
An official website of the United States government
The .gov means it’s official. Federal government websites often end in .gov or .mil. Before sharing sensitive information, make sure you’re on a federal government site.
The site is secure. The https:// ensures that you are connecting to the official website and that any information you provide is encrypted and transmitted securely.
- Publications
- Account settings
- My Bibliography
- Collections
- Citation manager

Save citation to file
Email citation, add to collections.
- Create a new collection
- Add to an existing collection
Add to My Bibliography
Your saved search, create a file for external citation management software, your rss feed.
- Search in PubMed
- Search in NLM Catalog
- Add to Search
A systemic review on tuberculosis
Affiliations.
- 1 Department of Microbiology, Sri Devaraj Urs Medical College, Sri Devaraj Urs Academy of Higher Education and Research, Kolar, India.
- 2 Department of Microbiology, S Nijalingappa Medical College, Bagalkot, India.
- 3 SDM Narayanaya Heart Centre, Sri Dharmasthala Manjunatheshwara Medical College, Sri Dharmasthala Manjunatheshwara University, Dharwad, India. Electronic address: [email protected].
- PMID: 32825856
- DOI: 10.1016/j.ijtb.2020.02.005
Tuberculosis (TB), which is caused by bacteria of the Mycobacterium tuberculosis complex, is one of the oldest diseases known to affect humans and a major cause of death worldwide. Tuberculosis continues to be a huge peril disease against the human population and according to WHO, tuberculosis is a major killer of the human population after HIV/AIDS. Tuberculosis is highly prevalent among the low socioeconomic section of the population and marginalized sections of the community. In India, National strategic plan (2017-2025) has a national goal of elimination of tuberculosis by 2025. It requires increased awareness and understanding of Tuberculosis. In this review article history, taxonomy, epidemiology, histology, immunology, pathogenesis and clinical features of both pulmonary tuberculosis (PTB) and extra-pulmonary tuberculosis (EPTB) has been discussed. A great length of detailed information regarding diagnostic modalities has been explained along with diagnostic algorithm for PTB and EPTB. Treatment regimen for sensitive, drug resistant and extensive drug resistant tuberculosis has been summarized along with newer drugs recommended for multi drug resistant tuberculosis. This review article has been written after extensive literature study in view of better understanding and to increase awareness regarding tuberculosis, as a sincere effort that will help eliminate tuberculosis off the face of the earth in near future.
Keywords: Immunology; Tuberculosis diagnosis; Tuberculosis pathogenesis; Tuberculosis treatment.
Copyright © 2020 Tuberculosis Association of India. Published by Elsevier B.V. All rights reserved.
PubMed Disclaimer
Similar articles
- History of Pulmonary Tuberculosis. Pezzella AT. Pezzella AT. Thorac Surg Clin. 2019 Feb;29(1):1-17. doi: 10.1016/j.thorsurg.2018.09.002. Thorac Surg Clin. 2019. PMID: 30454916 Review.
- The tenacity of tuberculosis. Aronson SM. Aronson SM. Med Health R I. 2008 Nov;91(11):331. Med Health R I. 2008. PMID: 19093378 No abstract available.
- Malignant tuberculosis. Saeed BW. Saeed BW. J Ayub Med Coll Abbottabad. 2006 Jul-Sep;18(3):1-2. J Ayub Med Coll Abbottabad. 2006. PMID: 17348302 No abstract available.
- Development of knowledge of unity of tuberculosis and of the portals of entry of tubercle bacilli. Myers JA. Myers JA. J Hist Med Allied Sci. 1974 Apr;29(2):213-28. doi: 10.1093/jhmas/xxix.2.213. J Hist Med Allied Sci. 1974. PMID: 4208667 No abstract available.
- The Evolutionary History, Demography, and Spread of the Mycobacterium tuberculosis Complex. Barbier M, Wirth T. Barbier M, et al. Microbiol Spectr. 2016 Aug;4(4). doi: 10.1128/microbiolspec.TBTB2-0008-2016. Microbiol Spectr. 2016. PMID: 27726798 Review.
- Paradoxical Reaction during Treatment of Tuberculous Meningoencephalitis in a Patient with Systemic Lupus Erythematosus. Versiani IL, Nimer GF, Moura CB, Xavier AF, Gaudio RC, Lopes FCR, Braune CB. Versiani IL, et al. J Glob Infect Dis. 2024 Jun 26;16(2):73-75. doi: 10.4103/jgid.jgid_96_23. eCollection 2024 Apr-Jun. J Glob Infect Dis. 2024. PMID: 39081506 Free PMC article.
- The impact of COVID-19 on tuberculosis in most populated state of India: A geospatial meta-analysis. Priyadarshi V, Tripathi S. Priyadarshi V, et al. J Family Med Prim Care. 2024 Jul;13(7):2576-2584. doi: 10.4103/jfmpc.jfmpc_1970_23. Epub 2024 Jun 28. J Family Med Prim Care. 2024. PMID: 39071010 Free PMC article. Review.
- The Role of Diffusion Tensor Imaging in CNS Tuberculosis. Saurya S, Sharma G, Saxena BS, Gupta PK. Saurya S, et al. Cureus. 2024 Jun 23;16(6):e62998. doi: 10.7759/cureus.62998. eCollection 2024 Jun. Cureus. 2024. PMID: 39050293 Free PMC article.
- Diabetes and Infectious Diseases with a Focus on Melioidosis. Uthaya Kumar A, Ahmad Zan M, Ng CL, Chieng S, Nathan S. Uthaya Kumar A, et al. Curr Microbiol. 2024 Jun 4;81(7):208. doi: 10.1007/s00284-024-03748-z. Curr Microbiol. 2024. PMID: 38833191 Review.
- Determinants of sputum culture conversion time in multidrug-resistant tuberculosis patients in ALERT comprehensive specialized hospital, Addis Ababa, Ethiopia: A retrospective cohort study. Abebe M, Atnafu A, Tilahun M, Sero N, Neway S, Alemu M, Tesfaye G, Mihret A, Bobosha K, Wan C. Abebe M, et al. PLoS One. 2024 May 31;19(5):e0304507. doi: 10.1371/journal.pone.0304507. eCollection 2024. PLoS One. 2024. PMID: 38820260 Free PMC article.
Publication types
- Search in MeSH
LinkOut - more resources
Full text sources.
- Elsevier Science
- Genetic Alliance
- MedlinePlus Health Information
- Citation Manager
NCBI Literature Resources
MeSH PMC Bookshelf Disclaimer
The PubMed wordmark and PubMed logo are registered trademarks of the U.S. Department of Health and Human Services (HHS). Unauthorized use of these marks is strictly prohibited.
- Journal club
- Subscriptions
- Advanced search

Advanced Search
New developments in tuberculosis diagnosis and treatment
- Find this author on Google Scholar
- Find this author on PubMed
- Search for this author on this site
- ORCID record for Cara M. Gill
- For correspondence: [email protected]
- Figures & Data
- Info & Metrics
Tuberculosis (TB) is a major cause of morbidity and mortality worldwide. It is estimated that 25% of the world's population are infected with Mycobacterium tuberculosis , with a 5–10% lifetime risk of progression into TB disease. Early recognition of TB disease and prompt detection of drug resistance are essential to halting its global burden. Culture, direct microscopy, biomolecular tests and whole genome sequencing are approved methods of diagnosis; however, their widespread use is often curtailed owing to costs, local resources, time constraints and operator efficiency. Methods of optimising these diagnostics, in addition to developing novel techniques, are under review. The selection of an appropriate drug regimen is dependent on the susceptibility pattern of the isolate detected. At present, there are 16 new drugs under evaluation for TB treatment in phase I or II clinical trials, with an additional 22 drugs in preclinical stages. Alongside the development of these new drugs, most of which are oral medications, new shorter regimes are under evaluation. The aim of these shorter regimens is to encourage patient adherence, and prevent relapse or the evolution of further drug resistance. Screening for TB infection, especially in vulnerable populations, provides an opportunity for intervention prior to progression towards infectious TB disease. New regimens are currently under evaluation to assess the efficacy of shorter durations of treatment in this population. In addition, there is extensive research into the use of post-exposure vaccinations in this cohort. Worldwide collaboration and sharing of expertise are essential to our ultimate aim of global eradication of TB disease.
Educational aims
Differentiate between TB infection and TB disease.
Understand the different methods of diagnosing TB disease and resistance.
Recognise the different drugs and regimens currently in use for TB disease.
Be able to discuss risk of TB disease in TB infection, and assist patients in making an informed decision on treatment for TB infection.
Early detection of drug resistance is essential to our goal of global eradication of TB. Tolerable drugs and shorter regimens promote patient adherence. Treating TB infection in vulnerable groups will prevent further global spread of TB disease. https://bit.ly/3oUW0SN
- Introduction
Tuberculosis (TB) is a major cause of morbidity and mortality worldwide. TB is caused by the bacillus Mycobacterium tuberculosis (Mtb ), which is spread via airborne droplets. Approximately one in four people worldwide demonstrate an immunological response to Mtb infection, which can remain dormant or progress into active disease forms [ 1 ]. Patients infected with TB who have no active signs or symptoms of disease were previously deemed to have latent TB, more recently changed to TB infection [ 2 ]. Whereas patients with active disease are termed to have TB disease. Patients with TB infection have a 5–10% lifetime risk of developing TB disease, which increases in varying states of immunodeficiency up to a 16% annual risk of activation of TB infection into TB disease in HIV patients [ 3 ]. In 2019, there were an estimated 10 million new incident cases of active TB disease worldwide [ 1 ]. Approximately two-thirds of all cases arise in eight countries alone, the vast majority of which have overwhelmed health services with limited resources [ 1 ]. This significant global burden of disease has been recognised by the World Health Organization (WHO) who launched the End TB initiative in 2016. Their aim is to reduce incidence, morbidity and mortality of this disease by improving diagnostic and therapeutic practices, as well as developing preventative strategies, through innovative research and education. By 2035, the goal is to reduce TB mortality by 95% and reduce overall incidence of TB by 90% worldwide [ 4 ]. Owing to the work of our predecessors, it has been estimated that 60 million lives have been saved globally in the 21st century so far [ 5 ].
Effective TB treatment is dependent on:
Prompt diagnosis of TB and recognition of drug resistance;
Promoting and ensuring patient adherence to regimens;
Robust contact tracing and prophylactic treatment of contacts; and
Screening for TB infection in high-risk groups.
There is ongoing extensive research into developing accurate, timely methods of detecting drug resistance, even in resource poor settings. Many effective, less toxic medications are under development. Furthermore, methods of promoting and ensuring drug adherence are being reviewed. In addition, there is vital research ongoing in proactive areas of TB prevention, such as screening for, and treatment of, TB infection and developing efficacious vaccines to halt the spread of this killer disease.
The aim of this article is to: review current practice in the diagnosis and treatment of TB; outline new diagnostic techniques under development; discuss new drug therapies and treatment regimens under review; and review the evidence for vaccination.
Improving the efficiency and accuracy of TB diagnosis contributes to treatment efficacy. Pulmonary TB should be suspected when patients present with classical symptoms such as non-resolving cough, haemoptysis, fevers, night sweats and weight loss. Extrapulmonary TB, including TB lymphadenitis, TB meningitis, laryngeal TB, Pott's disease and abdominal TB, presents in a variety of manners. Special consideration should always be given to patients who have potential TB exposure, as well as immunocompromised patients who may present atypically. The diagnosis must be made by confirming the presence of the causative pathogen, Mtb . A variety of methods are employed to confirm the diagnosis. In addition, it is essential that there is emphasis on early detection of potential drug resistance.
Drug resistance is a growing issue that threatens TB care worldwide. Traditionally this was categorised into rifampicin-resistant TB (RR-TB), multidrug-resistant TB (MDR-TB) or extensively drug-resistant TB (XDR-TB). MDR-TB is resistant to both rifampicin (RIF) and isoniazid (INH). Recently definitions have been updated to include pre-XDR-TB, which is TB that fulfils the definition for MDR-TB and RR-TB that is also resistant to any fluoroquinolone (FLQ). The updated definition for XDR-TB is strains that fulfil the definition for MDR-TB/RR-TB which are also resistant to any group A drug (namely levofloxacin (LFX), moxifloxacin (MFX), bedaquiline (BDQ) and linezolid (LZD)) [ 6 ]. Replacing the old XDR-TB definition referencing second-line injectable drugs (SLID), it highlights the trend towards use of oral regimens comprising recently developed or repurposed drugs. Despite the importance of early recognition, only 61% of patients with a new diagnosis of bacteriologically confirmed TB disease in 2019 were tested for RIF resistance [ 1 ]. This is in part related to access to diagnostics in resource-limited settings. There are numerous methods currently available, and under development, to determine drug resistance. For these diagnostics to be beneficial on a global scale they need to provide timely, accurate, cost-effective results in centres where access to power, equipment and technical expertise remains limited.
Culture of Mtb in a suitable medium remains the gold standard diagnostic test. The specimen can be cultured in solid ( e.g. Löwenstein–Jensen or Middlebrook 7H11) or liquid media ( e.g. for use with the BACTEC Mycobacterium Growth Indicator Tube (MGIT) 960 system). Sensitivity, specificity, contamination rates and time to detection vary widely amongst both media, with the WHO advocating for dual use of systems where practical. The major benefit of the advent of liquid-based systems is the rapid time to detection, often reducing time to growth by half with a mean time to detection of 12.8 days compared with 25.1–25.5 days for the previously mentioned solid media [ 7 ]. However, sub-optimal laboratory facilities in resource-limited settings often restrict its practical use [ 8 ]. While culture is not recommended for use as a first-line test, it remains an important part of TB diagnostics where persistent culture positivity can predict likelihood of relapse [ 9 ].
Direct microscopy
Direct microscopy is a fast and inexpensive method to identify acid-fast bacilli (AFB), the majority of which are mycobacteria [ 10 ]. Traditionally, Ziehl–Neelsen (ZN) stain was applied and the sample termed “smear positive” or “smear negative”, depending on the presence or absence of AFB. Efficacy is operator dependent, resulting in a broad range of sensitivities and specificities reported in international studies, 25.3–81.6% and 83.4–99%, respectively [ 11 , 12 ]. It is even less sensitive in high-risk groups, such as patients with HIV, and children [ 1 ]. Methods to improve efficacy include use of mercury vapour fluorescence and light-emitting diode (LED) microscopy, which have largely replaced traditional ZN staining [ 13 ]. Education and quality assurance for laboratory technicians is one of the most useful ways to ensure accurate diagnosis, as direct microscopy often remains the only method of diagnosis available in resource-limited settings [ 14 ]. Similar to culture, direct microscopy remains an integral part of monitoring response to treatment, measuring infectiousness, and predicting likelihood of relapse in patients who are smear positive at diagnosis.
Molecular tests
Given the limitations of culture and direct microscopy, the WHO recommends a biomolecular test as the initial diagnostic test in a suspect patient [ 1 ]. Current molecular tests endorsed by WHO include: Xpert MTB/RIF and Xpert MTB/RIF Ultra assays (Cepheid, Sunnyvale, USA); loop-mediated isothermal amplification test (TB-LAMP; Eiken Chemical, Tokyo, Japan); Truenat MTB, MTB Plus and MTBRIF Dx tests (Molbio Diagnostics, Goa, India) and lateral flow urine lipoarabinomannan assay (LF-LAM; Alere Determine TB LAM Ag, Abbott, San Diego, USA).
The WHO currently recommends Xpert (MTB/RIF or MTB/RIF Ultra) or Truenat (MTB or MTB Plus) as the initial diagnostic test of choice in suspected pulmonary TB [ 1 ]. They are cartridge based nucleic acid amplification tests (NAAT) that detect the presence of TB DNA, as well as common mutations associated with RIF resistance along the rpoB gene, within 2 h [ 15 ]. The Xpert MTB/RIF and Xpert MTB/RIF Ultra assays are also endorsed by the WHO for diagnosing extrapulmonary TB and TB in children [ 1 ]. When compared with culture diagnosis, the Xpert assays have demonstrated 89% sensitivity and 99% specificity at diagnosing pulmonary TB in adults [ 16 ]. The Xpert MTB/RIF Ultra assay has a higher sensitivity but lower specificity than the Xpert MTB/RIF assay, owing to its inability to accurately differentiate between dormant and active TB DNA [ 17 , 18 ]. While recommended for use, it is important to remember these assays have reduced sensitivity in certain populations such as children and patients coinfected with HIV, as well as in extrapulmonary TB [ 16 , 19 ]. Moreover, this technology is expensive and requires laboratory facilities with continuous access to power. To overcome this obstacle in resource-limited settings, there are a number of smaller, battery-operated technologies in development. To date, the GeneXpert Omni (Omni; Cepheid) appears to be the most promising potential candidate for widespread use. In a real-world analysis, it has been shown to be a cost-effective method when used in peripheral healthcare settings [ 20 ]. It allows diagnosis to be at/near the point of care, and thus avoids further delays and costs associated with transporting samples to specialised centres.
As well as the Omni, Cepheid is also developing the Xpert MTB/XDR assay. It aims to also detect resistant to INH, FLQ, ethionamide (ETH) and SLID. Similar to other Xpert assays, it is a NAAT that detects 16 clinically relevant mutations associated with resistance in under 90 min [ 21 ]. When compared with phenotypic drug sensitivity testing (pDST), it has a 94% sensitivity and 100% specificity at detecting drug resistance [ 21 ]. There are large scale multicentre clinical trials ongoing to establish its real-world efficacy as a follow-on test to current Xpert MTB/RIF and MTB/RIF Ultra assays, prior to consideration for WHO recommendation. This assay is of paramount importance as the early recognition of drug resistance is a prerequisite to shorter drug regimens, which will be discussed in further detail elsewhere in this review.
While most biomolecular tests are NAAT detecting the presence of Mtb DNA, the LF-LAM test detects a lipopolysaccharide present in mycobacterial cell walls. While not in use in most countries in the developed world, the LF-LAM assay has been recommended for use in HIV-coinfected patients. It is a urinary antigen test that is often employed in resource-limited settings, and is of particular benefit in cases where a sputum sample cannot be obtained. It has a 42% sensitivity in HIV patients with TB symptoms [ 22 ]. However, it cannot distinguish between mycobacterial species, and can cross react with other fungal diseases. As such, it is used as an initial test in peripheral primary care centres in areas of high TB endemicity only, to determine whether symptomatic patients with HIV should be referred for further confirmatory testing [ 23 ].
Line probe assays
Another method of molecular detection of Mtb resistance is line probe assay (LPA). Genotype MTBDR plus and Genotype MTBDR sl (Hain LifeScience GmbH, Nehren, Germany) are used for the detection of Mtb and its associated drug resistance. The WHO approved Genotype MTBDR plus employs a series of steps to detect Mtb and mutations in rpoB and katG , which confer RIF and INH resistance, respectively [ 24 ]. Additionally, it can detect the presence of inhA promoter genes that confer resistance to low dose INH, which are also typically associated with ETH and prothionamide resistance [ 25 ]. This in vitro test delivers results in <6 h [ 26 ]. When compared with traditional culture-based drug sensitivity, it is 78.5% sensitive and 100% specific at detecting RIF and INH resistance [ 27 ]. The WHO endorsed Genotype MTBDR sl 2.0 assay can also detect resistance conferring mutations of FLQ ( gyrA and gyrB ) and SLID ( rrs and eis ) [ 28 ]. Reported sensitivity and specificity are 100% and 98.9% for FLQ, and 89.2% and 98.5% for SLID [ 29 ]. Even more sensitive than NAAT at detecting FLQ resistance, this rapid test could allow for use of FLQ in patients that might otherwise have faced a lengthier regime that potentially required the interim use of SLID. However, these tests are not without limitations including low sensitivity for detecting ethambutol (ETM) and aminoglycoside resistance as demonstrated in a real-world analysis [ 30 ]. Similar in aim to the Xpert MTB/XDR assay, these LPAs provide prompt recognition of drug resistance, so patients can be started on the appropriate regimen and further drug resistance does not have an opportunity to develop while awaiting standard culture-based susceptibility results, nor are patients exposed to burdensome, longer drug regimens with higher potential for toxicity.
Whole genome sequencing (WGS)
While NAAT and LPA tests are rapid, accessible diagnostics, their efficacy at detecting drug resistance is hindered by the inability to detect clinically relevant mutations outside the rifampicin resistance determining region (RRDR) of the rpoB gene [ 31 ]. While 95% of resistant cases arise from mutations in this region, there have been a number of public health crises emerging from missed diagnosis of outbreaks that have arisen from mutations outside it [ 32 ]. One such example is the I491F mutation that has been responsible for an outbreak of MDR-TB in Eswatini and remains a grave public health concern [ 33 ]. Another limitation is the inability to differentiate silent mutations from those that hinder drug efficacy, thus delivering a higher rate of false positive resistance results [ 34 ]. WGS provides a comprehensive review of the entire Mtb genotype with a 96% concordance for culture-based sensitivity testing [ 35 ]. It provides genotypic sensitivity to most drugs required for treatment of MDR-TB [ 36 ]. While full clarification on clinical correlation between genotypic and phenotypic sensitivities remains to be shown, progress has been made in assigning probability of pDST based on genotypic results [ 37 ]. Utility was initially limited in low-income countries by cost and requirement for robust facilities and technical expertise [ 38 ]. However, with ongoing technological advancements in the microfluidic approaches to TB diagnosis, WGS is likely to be available at point of care on a global basis [ 39 ]. For some countries, it remains an important tool not only in case diagnosis, but in formulating public health policy by assisting in tracing TB contact cases in outbreaks [ 40 ]. In the future, with improved knowledge of the genomics involved in TB resistance, WGS is likely to prove revolutionary in tailoring TB treatment to each individual patient based on the particular genome identified by the Mtb strain they have contracted.
Culture-based drug sensitivity testing (DST)
As previously mentioned, the major advantage of liquid culture is rapidity of growth, which has led to more widespread use of liquid broth-based methods such as the MGIT. BACTEC MGIT 960 is a fully automated system that delivers results within 2 weeks [ 41 ]. Culture-based DST remains the gold standard for determining drug resistance at present [ 1 ]. The two approaches currently in use are the critical concentration and minimum inhibitory concentration (MIC). Classically, critical concentration was defined as the lowest concentration of a drug that inhibits growth of 95% of Mtb strain present. Owing to ongoing research, these critical concentrations are regularly updated with a recent reduction in the critical concentration required to determine RIF resistance, allowing for greater concordance between genotypic and phenotypic sensitivity results [ 42 ]. Alternatively, the MIC method is defined as the lowest concentration of a drug that results in complete inhibition of visual growth of the Mtb strain in vitro . Following extensive work completed by national reference laboratories, and international discussion and agreement, a new reference MIC protocol has been set and validated by European consortia [ 43 ].
Computer aided detection for chest radiographs
Given the limitations, in terms of time, cost and infrastructure, to the above testing methods, it has become clear that there need to be affordable, accessible methods of screening available in high-burden areas to assist with risk stratification for allocating further testing. One such proposed method is the use of computer software to digitally interpret chest radiographs, and assign a score indicating the likelihood of TB. The most commonly studied software is CAD4TB, currently on version 6. When compared with NAAT, CAD4TB has been shown to have 90–100% sensitivity, and 23–45% specificity at detecting TB disease [ 44 ]. It performs similarly to expert clinicians and radiologists, with similar pitfalls including disease obscured by musculoskeletal findings and differentiating old scarring from new disease. Its use is intended for high-burden areas, that may lack readily accessible radiological expertise on site to interpret chest radiographs in a timely fashion [ 45 ]. It may assist peripheral health centres to determine which patients require further molecular testing.
Serum biomarkers
Another potential method for triage testing is serum biomarkers. Devising an accurate biomarker that upholds sensitivity across different ethnicities, HIV status and site of TB has proven difficult. However, a nine protein biosignature has recently been discovered which appears to remain efficacious in all of these cohorts. Using fibrinogen, α 2 -macroglobulin, C-reactive protein, matrix metalloproteinase-9, transthyretin, complement factor H, interferon-γ, interferon-γ inducible protein-10 and tumour necrosis factor-α as a host biosignature demonstrated 92% sensitivity and 72% specificity for determining TB from other diseases [ 46 ]. If available on a commercial level, this serum assay could rapidly and effectively determine which patients warranted further testing. It is important to note that most of these biomarkers are markers of inflammation, and as such are widely variable amongst patients and their differing metabolic and disease states. Evaluating serum biomarkers as predictors of response to treatment, potential for relapse and predictors of TB infection versus active disease will be discussed elsewhere.
Alongside research into obtaining accurate and timely diagnostics, there is tremendous work ongoing in developing safe, efficacious, tolerable treatment regimens. The goals of treatment are not only to eradicate disease, but to prevent long-term morbidity arising from either the disease itself or as an adverse effect of the drugs in use. Successful treatment of drug-sensitive TB (DS-TB) has been reported in 85% of patients [ 1 ]. Efficacy in drug-resistant forms is lower at 57% and is likely multifactorial [ 1 ]. To reflect this, there has been a trend towards oral drug regimens, where possible, given research highlighting patient preference and cost-effectiveness of these drugs [ 47 ]. We need to deliver a regimen that will not only aid our global goal of TB eradication, but in a manner that reflects our patients’ wishes, and in doing so, promotes their compliance.
Current treatment
The current medications approved for use in TB treatment, and their notable side-effects are summarised in table 1 .
- View inline
Current medications in use for TB treatment and their notable side-effects
DS-TB tends to follow a standard 6-month regime. This comprises an intensive phase with 2 months treatment consisting of RIF, INH, pyrazinamide (PZA) and ETM, followed by a continuation phase with 4 months treatment of RIF and INH [ 48 ]. If the isolate is susceptible to both RIF and INH, ETM can be stopped. The continuation phase should be extended to 7 months in the presence of: cavitation on the initial chest radiograph; persistent sputum growth at 2 months; or if PZA cannot be used due to monoresistance or drug side-effects. Consideration should also be given to extending this phase to 7 months in patients who are otherwise immunosuppressed, such as patients with HIV, diabetes mellitus, malignancy or medications associated with immunosuppression [ 48 ]. Unfavourable outcomes are most associated with high grade smear positivity (at least 3+) and dependent on the size of cavities, as well as extent of disease on chest radiographs [ 49 ].
Current treatment of drug-resistant TB is more complex and is summarised in table 2 . Most notable is the longer duration of treatment involving combinations of drugs that are often poorly tolerated. There is also minor discordance between the two major international advisory bodies (the WHO and the joint ATS/CDC/ERS/IDSA clinical practice guideline) concerning optimum drug selection and durations. While the WHO recommends only four drugs need to be used in the intensive phase of treatment, the ATS/CDC/ERS/IDSA propose continuing to use five drugs in this phase. The ATS/CDC/ERS/IDSA have proposed this recommendation based on higher success rates in the five-drug group (93.9% versus 89.7%; adjusted odds ratio (aOR) 3.0 versus 1.2; risk difference 8% in both groups). Additionally, they suggest it is likely that one of the drugs may need to be withdrawn due to toxicity [ 50 ]. However, given equivocal risk differences in both groups, the WHO maintain four drugs should be sufficient, providing susceptibilities are known and toxicity is unlikely. De-escalation to a continuation phase comprising three or four drugs is based on similar evidence. Traditionally, MDR-TB required treatment for a total duration of 15–21 months [ 50 ]. Alternatively, it does allow for a shorter 9–12 month all oral regimen for patients who have not previously had more than 1 month of treatment with second-line medications, and in whom FLQ resistance has been ruled out. Additionally, patients should not have extensive disease [ 51 ]. This shorter regimen involves 4 months of six drugs (FLQ, clofazimine (CFZ), ETH, PZA, INH (high dose)), followed by 5 months of FLQ, CFZ, ETH and PZA. BDQ is used concurrently for the first 6 months of this regimen. This conditional recommendation of low certainty evidence was proposed owing to improved success and adherence rates, when compared with shorter regimens containing injectable agents (aOR 1.9 (95% CI 1.6–2.4)) [ 52 ]. (Note, INH is used regardless of susceptibility status).
Current ATS/CDC/ERS/IDSA consolidated guidelines on treating drug-resistant TB
At present, the WHO recommends treatment for RR-TB in line with MDR-TB.
Pre-XDR- and XDR-TB are more difficult to treat, owing to varying patterns of drug resistance and advice should always be sought from national and international expert TB consortia prior to commencing treatment.
New treatment: drugs
At present, there are 16 new drugs in phase I or II clinical trials, and 22 other drugs in discovery or preclinical phases of development, as outlined in figure 1 . Of those drugs undergoing clinical trial, there are 11 drugs of new chemical classes. Of the remaining drugs, TBAJ-587 and TBAJ-876 are diarylquinolines, similar to BDQ, while delpazolid, sutezolid and TBI-223 are oxazolidinones, similar to LZD and cycloserine. At the time of publication, no new drugs have reached phase III trials or been approved for market regulation since the approval of pretomanid (Pa) in 2019. A promising candidate from a new drug class is telacebec. It induces bacterial cell death by inhibiting the mycobacterial cytochrome bc1 complex responsible for ATP synthesis. A proof-of-concept trial has shown increased rates of sputum clearance, with comparable levels of adverse events to currently approved drugs. If results from ongoing clinical trials continue to reflect this, it is likely to be approved as a third new modern drug class with anti-tuberculous activity [ 53 ]. This would be an important achievement as many of the other drugs in development are classified similarly to existing drugs, and as such their use in additive or substitutive places for their relative counterparts will be precluded due to concerns regarding toxicity or resistance. It is also interesting to note that these drugs in development are largely oral preparations, owing to patient preference and thus potential for greater adherence and cure.
- Download figure
- Open in new tab
- Download powerpoint
New anti-tuberculous drugs currently in development. Data from [ 104 ].
New treatment: routes of delivery
While not a novel idea, interest in inhalation routes has been re-ignited. Numerous methods of drug delivery have been shown to be effective in animals, and additional advantages include reduced dosage and systemic toxicity. However, it would likely have no benefit on extrathoracic disease, nor would it be likely to achieve adequate therapeutic serum concentrations. Similar to the use of nebulised aminoglycosides in non- Mycobacterium tuberculosis , the potential for inhalational therapies to augment TB therapy likely lies as an adjunctive therapy to oral or injectable drug regimens. To date, there has been no data published from similar trials investigating its efficacy in sputum clearance from Mtb disease.
New treatment: regimes
In a disease that has the potential to affect one quarter of the world's population, it is astounding that no advances have been made in progressing the regimen for DS-TB since the mid-20th century. At present, international consensus guidelines continue to endorse standard 6-month regimes for the majority of cases of DS-TB, with varying longer regimens requiring expert opinion for drug-resistant cases. However, much research is being done into assessing shorter regimens with the aim of improving patient adherence and reducing risk of relapse and evolution of drug resistance, as seen in table 3 .
A shorter 4-month regimen of rifapentine (RFP) in combination with MFX has recently been shown to be non-inferior to the current standard 6-month regimen, as determined by negative smear or culture at 12 months, with no increase in major adverse events [ 54 ].
The SimpliciTB group have evaluated a 4-month regimen comprising BDQ, Pa, MFX and PZA, in place of the standard 6-month regimen. While it has been said that no drug should ever be kept in reserve, it is unlikely that this regimen will be recommended as first-line therapy for DS-TB, given the need to preserve efficacious drug options for resistant cases [ 55 , 56 ].
Shorter again with a 2-month regime, the TRUNCATE-TB trial is at recruitment phase. This multi-armed approach will assess combinations of 4–5 currently approved oral anti-tuberculous medications given daily for 8 weeks, with the potential to extend to 12 weeks [ 57 ].
The RIFASHORT and ReDEFINe studies are evaluating the risk–benefit ratio of higher doses of RIF in DS-TB [ 58 , 59 ]. The evidence base for these ongoing trials has been provided by the HIRIF trial which found an increased rate of sputum clearance, with no associated increase in toxicity, in patients on higher doses of RIF than currently recommended by the WHO [ 60 ].
2) Drug-resistant TB
• The current recommendations for RR-TB have been considered contentious for quite some time. These longer regimens likely expose patients with mono-resistance to unnecessarily long and toxic drug regimens, and also exclude the benefits of INH therapy [ 61 ]. BEAT TB is at the enrolment stage assessing the efficacy of 6 months of BDQ, LZD, delamanid (DLM), LFX and CFZ in comparison to current practices in South Africa [ 62 ].
• The updated STREAM2 study is evaluating a shorter regimen for RR-TB and MDR-TB in a simultaneous multi-armed approach. Their four regimens are based on: current WHO practice; the Bangladesh regime; a 40-week all oral regimen; and a 28-week oral regimen after an 8 week intense regime that also involves INH and kanamycin (Kan) [ 63 , 64 ].
• Results from the NEXT trial completed in December 2020 are awaited. This group compared 6–9 months of LZD, BDQ, LFX, PZA and ETH or INH (high dose) to current standards of care [ 65 ].
• TB-PRACTECAL stopped early due to superior outcomes in the intervention arm, consisting of a 6-month regimen of BDQ, Pa, LZD and MFX. Full results are awaited [ 66 , 67 ].
• SimpliciTB are also evaluating a regimen for RR-TB/MDR-TB consisting of the same drugs as the DS-TB protocol (BDQ, PZA, MFX, Pa) but for 6 months [ 56 ].
• DELIBERATE are completing a phase II safety trial reviewing the safety and pharmacokinetics of combined BDQ and DLM therapy. Given the updated consensus guidelines, these drugs will often be given together and it is essential we have an evidence base for potential harms that may arise throughout the course of treatment [ 68 ].
• endTB, run by Médecins Sans Frontières, are evaluating a multi-armed approach combining varying combinations of an all oral regimen for 39 weeks. Similar to the STREAM2 study, this is the only other multi-armed trial reviewing multiple combinations of novel drugs simultaneously [ 69 ].
• The ZeNix trial is the only trial, at present, that is reviewing treatment regimens for patients with pre-XDR- or XDR-TB. Using BDQ, Pa and either LZD (BPaL) or placebo for a total duration of 26 weeks, their aim is to assess rates of sputum conversion. This trial is also one of the few to follow patients for a significant period post-treatment, and patients with be reviewed for 78 weeks following the end of treatment [ 70 ]. Data from its predecessor the Nix-TB trial has shown 88% favourable outcomes at 24 months following treatment in patients with either MDR- or XDR-TB [ 71 ]. This BPaL regime can currently be used under operational research conditions in patients with MDR-TB, in accordance with WHO guidance [ 51 ].
New drug regimens under evaluation
While many of these trials demonstrate promise for an improved approach to TB treatment, it is essential that we see long-term data on their efficacy and relapse rates prior to implementing them on a global scale. The fear is that these patients may have excellent short-term results, but disease recurs soon after with the added potential for drug resistance to develop.
New treatment: adjuncts
In addition to shorter regimens, with new or re-purposed drugs, there is research into methods of modifying the host immune response to improve treatment outcomes and prevent permanent morbidity from TB disease. As previously discussed, upon infection with Mtb the host can either suppress bacillary replication into a latent state, or the host is overwhelmed and active disease develops [ 72 ]. Both deficient and hyperinflammatory states have been associated with TB disease morbidity and mortality, suggesting that tailoring a balanced immune response is of paramount importance to survival [ 73 ]. With evolving knowledge of the pathways and subcellular responses involved, new therapeutic targets are being developed to assist with bacillary quiescence in the so called “host directed therapy” approach [ 74 ]. Numerous drug targets have been suggested, largely centred on modulating macrophage activity [ 75 ]. Proposed adjunctive therapies include vitamin D, everolimus, auranofin and CC-11050, a novel anti-inflammatory compound. Preliminary results from trial data suggest none of these compounds improve rates of sputum conversion; however, patients in receipt of CC-11050 or everolimus had increased recovery of FEV 1 (forced expiratory volume in 1 s) post-treatment, perhaps solidifying the role of a balanced immune response to infection [ 76 ].
New treatment: the future
Going forward, with a combination of new drugs, altered durations and more effective testing of response to treatment, it is likely that each patient will have a tailored approach to TB treatment [ 49 ]. With studies like PredictTB, aiming to determine biomarkers and radiographic appearances that predict response and likelihood of relapse, we will be able to devise a drug combination and duration with greater specificity for each patient [ 77 ]. Similar technology may even assist with developing even more efficacious drugs in early-stage clinical trials [ 78 ]. Additionally, it is essential that any new drug or technology developed is affordable and available to all institutions, most importantly hospitals in low-resource environments, where the majority of the global TB burden persists.
Despite ongoing research, treatment for DS-TB has remained unchanged for decades. This highly effective regimen is often poorly tolerated by patients, and “drug holidays” are frequent during treatment. This, of course, increases the likelihood of relapse and evolution of drug resistance. Moreover, patients with resistant TB have to endure longer regimens with their own associated side-effects. While awaiting the development and approval of less toxic regimens, there are a number of measures we can take to ameliorate adverse effects of treatment and promote patient adherence. It has been shown that comprehensive patient-centred approaches, involving nutritional, financial and psychological support, have higher rates of completion. In addition, patients with increased contact with healthcare workers tended to have lower drop-out rates during treatment [ 79 ]. The evidence base for this is provided by systematic reviews of mostly observational case studies and case cohorts, and as such randomised research in this area is required to determine a formal link.
Directly observed therapy
Directly observed therapy (DOT) has been a standard of care in TB treatment for several years. The premise is that patients are more likely to comply if medication ingestion is witnessed multiple times per week. Current recommendations are that it should be implemented in MDR- or XDR-TB cases, or for patients with complex or vulnerable care needs, such as homelessness, comorbid psychiatric illness or addiction [ 80 ]. There have been conflicting results from systematic reviews on the efficacy of DOT [ 81 , 82 ]. What is known, is that community-based DOT appears to be the most effective strategy, as it is less disruptive for patients and thus their adherence is more likely to be maintained [ 83 ]. In recent years, attention has switched towards the use of smartphone technology. Video observed therapy (VOT) has been suggested as an even less disruptive form of monitoring adherence [ 84 ]. Patients can either upload videos of medication ingestion to a secure platform to be watched at a later date, or it can be taken while on a live feed with their healthcare team. VOT has been shown to have a higher uptake rate and patient preference rating [ 85 ]. While plausible that this will improve adherence, and thus relapse should be less likely, this study was not sufficiently powered to assess this, nor did it follow up on relapse rates at an appropriate interval. A real-world efficacy and cost-effectiveness study is ongoing in a tertiary hospital in Ireland at present [ 86 ].
- Prophylaxis
Undoubtedly, a burden of TB infection will persist for years to come. However, we have a chance to prevent many of these patients from progressing to active disease. Screening for TB infection in groups at high risk of progressing to TB disease remains a cost-effective and essential component to the global initiative. Screening via either of the endorsed interferon-γ release assays (QuantiFERON-TB Gold In-Tube and T-SPOT.TB) or traditional tuberculin skin testing is recommended in certain populations. The WHO has advised that clinical judgement is paramount in interpreting these tests, and cautions that a higher rate of false negatives occurs in the most vulnerable populations [ 87 ]. Another essential component of the sustainable development goals is robust public health policy to assist in contact tracing of index cases and early treatment of contacts. In addition, prior to any prophylactic treatment being commenced, it is essential that due caution is taken to rule out the presence of active TB disease.
Currently the WHO advocates for treatment with 4 months of RIF or 6–9 months of INH in cases where the index case is known to be drug sensitive [ 87 ]. A 3-month combination of RIF and INH is also approved, although rarely used due to potential toxicity. Additionally, weekly INH and RFP for 3 months has been shown to demonstrate equal efficacy and toxicity in comparison to 6 months of INH therapy, while higher levels of adherence were noted in the INH/RFP arm [ 88 ]. Moreover, a 1-month regimen of RFP/INH therapy was non-inferior to 9 months INH monotherapy in preventing TB in HIV-infected patients [ 89 ]. However, this regimen has yet to be endorsed by major international consortia.
The recommendations for TB contacts of DS-TB cases who demonstrate evidence of TB infection are as per those above. For contacts of MDR-TB cases, the current recommendation is for 6–12 months treatment with a FLQ with or without a second drug. If a FLQ cannot be used due to resistance in the index case, treatment with ETM and PZA is to be considered [ 87 ]. Regardless of the regimen in use, it is vital that strict adherence is maintained to ensure efficacy and prevent resistance.
At present, the decision to treat is based on the potential for progression to active disease based on similar case profiles. Going forward, we could vastly improve the cost efficacy of this intervention by being able to determine exactly which patients were going to progress to active TB disease or not. It had been hoped the answer would lie in serum transcriptional biomarkers and host response-based gene signatures [ 90 , 91 ]. Recently, a four-protein biomarker panel has shown 67.3% sensitivity and 96.3% specificity at determining active from latent TB [ 92 ]. This subclinical phase of TB disease can be difficult to interpret due to its lower inflammatory profile and person specific confounding factors that influence our immune response. Recent results from transcriptomic studies have been disappointing overall, but may potentially suggest a role for these panels in symptomatic patients with known TB infection and their risk of progression to TB disease in an imminent 6-month period [ 93 ].
- Vaccination
Given the current prevalence of TB infection, with the associated lifetime risk of progressing to active disease, it is paramount that we protect future generations from this burden by halting transmission entirely. With greater understanding of the cellular processes involved in Mtb susceptibility and pathogenesis, scientists have been able to identify various potential targets with a role in vaccination. Central to this is the cellular immune response, with a need to upregulate T-helper cell (Th)1, and downregulate Th2 and regulatory T-cell responses [ 94 ]. It appears that Mtb has also recognised the need to adapt to this hypo-inflammatory phenotype with more modern strains displaying shorter latency and higher virulence than previously seen [ 95 ].
The only worldwide approved vaccine against TB remains bacillus Calmette–Guérin (BCG), effectively reducing the risk of severe childhood disease from TB, with an 85% reduction in TB meningitis and miliary TB in those <10 years of age [ 96 ]. It has also been noted that infants innoculated with BCG have increased survival and lower rates of other childhood infections. This observation is likely secondary to BCG's ability to prime innate immunity through epigenetic modification of innate immune cells [ 97 ].
Vaccination can be categorised into preventive pre-exposure, preventive post-exposure or therapeutic [ 98 ]. Vaccines can alternatively be classified according to their biochemical forms: live attenuated, inactivated, protein subunit or recombinant [ 99 ]. With each of these forms, the aim is to target various cells or subcellular components of TB pathogenesis.
MTBVAC, a pre-exposure live attenuated vaccine, has shown promising results from preclinical trials with a higher protection against TB than BCG [ 100 ]. This live vaccine is based on a genetically modified mutant Mtb strain containing deletions in transcription factors important for Mtb growth in macrophages and subsequent virulence.
VPM1002, another live recombinant BCG vaccine, is undergoing phase III studies at present to evaluate its efficacy at not only preventing infection, but in preventing active disease in those already affected [ 101 ]. This vaccine can modify T-cell immune response and enhance Th1 immunity, important in TB disease pathogenesis.
Another promising post-exposure candidate is M72/AS01E, a subunit vaccine, that prevents pulmonary TB in adults already infected with Mtb in 54% of patients, and thus could be a potentially life-saving intervention for one quarter of the world's population [ 102 ]. Also known as Mtb72F this vaccine comprises two immunogenic proteins that promote T-cell proliferation and interferon-γ release [ 103 ].
Further randomised control trials are warranted in a timely manner if the END TB strategy is to be achieved.
The future is bright for TB treatment. Never before has there been such a global effort to develop new technologies and treatment for TB patients. Combining these advancements, it is possible that we will base each patient's treatment on their own protein biosignatures in conjunction with the genomic expression of mutations in the Mtb strain they have been affected with. If we are to achieve our goal of global eradication of TB, it is essential that we continue to collaborate and share our expertise on an international scale to ensure each patient gets the appropriate treatment and support to overcome their TB diagnosis without significant morbidity.
Self- evaluation questions
1. What proportion of the world's population are estimated to have TB infection ( i.e. demonstrate immunological evidence of prior TB exposure)?
2. Which of the following is a WHO recommended first-line test in the diagnosis of TB disease?
b) Biomolecular test ( e.g. Xpert MTB/RIF or Truenat MTB)
c) Line probe assay ( e.g. Genotype MTBDR plus )
d) Serum interferon-γ release assay
3. Pre-XDR-TB is defined as TB that is resistant to rifampicin, isoniazid and what other drug(s)?
a) Linezolid
b) Second-line injectable drugs ( e.g. amikacin)
c) Fluoroquinolones ( e.g. moxifloxacin)
d) Bedaquiline
4. Which of the following regimens are not currently recommended by the WHO for preventive treatment of TB disease ( i.e. treatment of TB infection)?
Rifampicin (10 mg·kg −1 up to 600 mg maximum) daily for 4 months
Isoniazid (5 mg·kg −1 up to 300 mg maximum) daily for 6–9 months
Rifampicin (10 mg·kg −1 up to 600 mg maximum) and Isoniazid (5 mg·kg −1 up to 300 mg maximum) daily for 3 months
Rifapentine (900 mg if weight >50 kg, adjusted if less) and Isoniazid (15 mg·kg −1 up to 900 mg maximum) weekly for 1 month
Suggested answers
Conflict of interest: C.M. Gill has nothing to disclose.
Conflict of interest: L. Dolan has nothing to disclose.
Conflict of interest: L.M. Piggott has nothing to disclose.
Conflict of interest: A.M. McLaughlin has nothing to disclose.
- Received October 1, 2021.
- Accepted November 16, 2021.
- Copyright ©ERS 2022
Breathe articles are open access and distributed under the terms of the Creative Commons Attribution Non-Commercial Licence 4.0.
- World Health Organisation
- Selwyn PA ,
- Lewis VA , et al.
- Uplekar M ,
- Lonnroth K , et al.
- Centers for Disease Control and Prevention
- Drancourt M
- Gooze L , et al.
- Sotgiu G , et al.
- Sharma PP , et al.
- Ga S-P , et al.
- Singhal R ,
- Lumb R , et al.
- Bates M , et al.
- Steingart KR ,
- Schiller I ,
- Horne DJ , et al.
- Dorman SE ,
- Schumacher SG ,
- Alland D , et al.
- van Soolingen D
- Kendall E , et al.
- Gaur RL , et al.
- Bjerrum S ,
- Dendukuri N , et al.
- Streicher EM ,
- Hoek KG , et al.
- Somoskovi A ,
- Dormandy J ,
- Rivenburg J , et al.
- Kuo YM , et al.
- HAIN LifeScience
- Dreyer AW ,
- Koornhof HJ , et al.
- Pleń M , et al.
- Lau TC , et al.
- Sanchez-Padilla E ,
- Beckert P , et al.
- Palacios JJ ,
- Herranz M , et al.
- Nikolayevskyy V ,
- Kranzer K ,
- Niemann S , et al.
- McNerney R ,
- Preston MD , et al.
- Allix-Béguec C ,
- Arandjelovic I , et al.
- CRyPTIC Consortium and the 100,000 Genomes Project
- Eddabra R ,
- Ait Benhassou H
- Harrison J ,
- McGrath JS , et al.
- Roycroft E ,
- Fitzgibbon MM ,
- Kelly DM , et al.
- Tortoli E ,
- Cichero P ,
- Piersimoni C , et al.
- Werngren J ,
- Machado D , et al.
- Jeagal L , et al.
- Zaidi SMA , et al.
- Morris TC ,
- Hoggart CJ ,
- Chegou NN , et al.
- Migliori GB ,
- Zumla A , et al.
- Alipanah N , et al.
- Imperial MZ ,
- Phillips PPJ , et al.
- Migliori GB , et al.
- Mirzayev F ,
- Linh NN , et al.
- de Jager VR ,
- van Niekerk C , et al.
- Kurbatova EV , et al.
- ClinicalTrials.Gov
- Velasquez GE ,
- Brooks MB ,
- Coit JM , et al.
- Malenfant JH ,
- Van Deun A ,
- Salim MA , et al.
- Medecins San Frontieres
- Conradie F ,
- Ngubane N , et al.
- Oh SF , et al.
- Palucci I ,
- Whitworth LJ ,
- Redmond S , et al.
- Wallis RS ,
- Ginindza S ,
- Beattie T , et al.
- Daftary A ,
- O'Donnell M , et al.
- National Institute of Health and Care Excellence
- Volmink J ,
- Bachmann MO , et al.
- Garfein RS ,
- Hayward A , et al.
- Aldridge RW ,
- Smith CM , et al.
- Health Innovation Hub Ireland
- Sterling TR ,
- Villarino ME ,
- Borisov AS , et al.
- Chaisson RE ,
- Ramchandani R ,
- Swindells S
- Warsinske H ,
- Vashisht R ,
- Cobelens F ,
- Liu P , et al.
- Scriba TJ ,
- Fiore-Gartland A ,
- Penn-Nicholson A , et al.
- Mangtani P ,
- Abubakar I ,
- Ariti C , et al.
- Kleinnijenhuis , J ,
- Quintin J ,
- Preijers F , et al.
- Das G , et al.
- Whitlow E ,
- Mustafa AS ,
- Gonzalo-Asensio J ,
- Marinova D ,
- Martin C , et al.
- Nieuwenhuizen NE ,
- Kulkarni PS ,
- Shaligram U , et al.
- Ottenhoff THM
- Nabavinia MS ,
- Naderi Nasab M ,
- Meshkat Z , et al.
- Working Group on New TB Drugs

- Table of Contents
- Index by author
Thank you for your interest in spreading the word on European Respiratory Society .
NOTE: We only request your email address so that the person you are recommending the page to knows that you wanted them to see it, and that it is not junk mail. We do not capture any email address.
Citation Manager Formats
- EndNote (tagged)
- EndNote 8 (xml)
- RefWorks Tagged
- Ref Manager

- Respiratory infections and tuberculosis
- Tweet Widget
- Facebook Like
- Google Plus One
More in this TOC Section
- Management of mesothelioma
- Diagnostic pathology and molecular biomarkers in lung cancer
- The role of radiological imaging in severe and difficult-to-treat asthma
Related Articles
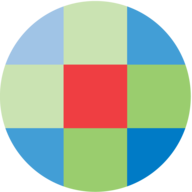
- Subscribe to journal Subscribe
- Get new issue alerts Get alerts
Secondary Logo
Journal logo.
Colleague's E-mail is Invalid
Your message has been successfully sent to your colleague.
Save my selection
Pulmonary tuberculosis
Improving diagnosis and management.
Singer-Leshinsky, Stacey MSEd, RPAC
Stacey Singer-Leshinsky is an associate professor at St. John's University in Queens, N.Y. The author has disclosed no potential conflicts of interest, financial or otherwise.
Earn Category I CME Credit by reading both CME articles in this issue, reviewing the post-test, then taking the online test at http://cme.aapa.org . Successful completion is defined as a cumulative score of at least 70% correct. This material has been reviewed and is approved for 1 hour of clinical Category I (Preapproved) CME credit by the AAPA. The term of approval is for 1 year from the publication date of February 2016.
Tuberculosis (TB) is a major public health concern worldwide and the world's second most common cause of death from infectious disease after HIV/AIDS. With the emergence of resistant strains of tuberculosis and increase in immunosuppressed patients, clinicians must be familiar with the clinical presentation of this potentially deadly infection. This article reviews the pathology, signs and symptoms, diagnostics, and management of TB, focusing on recent advances in drug therapy for drug-sensitive and drug-resistant forms. A better understanding of TB will help clinicians identify the disease early, reduce transmission, and provide treatment to prevent complications and reduce patient morbidity and mortality.

Pulmonary tuberculosis (TB) remains a major cause of morbidity and mortality worldwide, with about one-third of the world's population infected. 1 Between 10% and 20% of those infected will progress to active TB, posing a serious health threat. The remaining patients will have latent TB, which can advance to active infection in times of immunosuppression. The CDC reported 9,588 new cases of TB in the United States in 2013, a decline of 4.2% compared with 2012 and the lowest number of cases recorded in the United States since 1953. 2 However, TB continues to be a major health threat, especially among foreign-born persons. 2 Worldwide, more than 1 million patients with TB are coinfected with HIV, and of the more than 1.5 million TB-related deaths reported in 2011, most resulted from multidrug-resistant or extensively drug-resistant strains. 3 The increased prevalence of TB among HIV-infected patients, the evolution of multidrug-resistant strains, and a recent increase in international travel and immigration pose a serious threat to TB control. TB must be addressed more effectively in the United States to avoid a future epidemic of multidrug-resistant strains. 3
This article reviews the pathology, clinical presentation, and diagnosis of TB, focusing on advances in drug therapy. A better understanding of TB can help clinicians make accurate diagnoses and better manage this potentially deadly contagious disease.

PATHOGENESIS
TB is caused by the aerobic acid-fast rod-shaped bacterium Mycobacterium tuberculosis . 4 This tiny bacterium, expelled from a contagious host, can remain suspended in air as droplet nuclei for hours. 5 Speaking or a single cough or sneeze can generate many infective droplets, and as few as 10 bacilli can cause infection. 5 If inhaled by a susceptible host, Mycobacterium can lodge in the alveoli and are eventually taken up by alveolar macrophages; if they contain the disease, the patient has latent TB. If the macrophages fail to contain the disease, the patient develops active TB. The risk of developing active TB depends on the patient's age, immunocompetence, and time since infection. 6 Only patients with active disease are contagious.
Mucus-secreting goblet cells are the first line of defense, followed by alveolar macrophages of the innate immune system. The complement system assists in phagocytosis by producing protein C3, which binds to the bacterial cell wall, enhancing recognition and opsonization. 6 The macrophages produce proteolytic enzymes and cytokines that further degrade bacteria, and attract T cells that spark a cell-mediated immune response, releasing interleukin-12 and -18. 5 CD4 T lymphocytes release interferon gamma, resulting in further phagocytosis. 5 Interferon gamma leads to the release of tumor necrosis factor alpha and granuloma formation, limiting bacteria replication. In the center of the granuloma is a necrotic, caseating substance characterized by low oxygen levels, low pH, and limited nutrient supply. 6 Here the bacteria remain dormant and contained by an adequate host immune system until the granulomas undergo fibrosis and calcification. 7
The initial cell-mediated immune response takes 2 to 12 weeks to develop in a patient with a normal immune system, and is identified by a positive tuberculin skin test. About 45% of close contacts exposed to a contagious patient with active TB will become infected and have a positive T-cell-mediated hypersensitivity response. 8
About 5% of those infected will progress to active disease in the following 18 months of initial infection; the remaining 95% have a 5% risk of progressing to active disease in their lifetime. 4 Progression to active disease can occur if the patient's immune system becomes compromised by comorbidities such as HIV, diabetes, renal failure, malignancy, chronic steroid use, chemotherapy, the use of tumor necrosis factor inhibitors, or reinfection. Reactivation of latent TB accounts for about 70% of active TB cases. 9
A false-negative skin test result due to an impaired innate immune response is called anergy . Patients at risk for anergy are the young, older adults, and those with impaired immunity (such as in HIV infection). 1 In these patients, the initial infection can result in active primary TB because granuloma formation is suboptimal with loss of integrity. 8,10 Bacteria disseminate, leaving an air-filled fibrous cavity at the initial site of infection. 6 If bacteria enter the bronchus, they can be expelled via a cough, sneeze, or speaking, resulting in airborne droplet nuclei and contagious spread of the pathogen. Bacteria that drain into blood vessels can cause extrapulmonary forms of TB.
CLINICAL PRESENTATION
Active primary tuberculosis.
Despite TB's overt clinical presentation, confirmation is a challenge. Positive diagnostic findings are the only evidence of infection in patients with asymptomatic primary TB. Subclinical disease can present as paratracheal lymphadenopathy if bacteria have spread to the patient's lymphatic system. 6 As the primary lesion enlarges, and bacteria replicate, the patient will develop symptoms of respiratory distress (due to poor air exchange in affected tissue), chronic cough, crackles, sputum production, hemoptysis, pleuritic chest pain (due to inflamed parenchyma), fever, night sweats, and weight loss. 6 The clinical picture is nonspecific and the patient may be diagnosed with pneumonia, lung cancer, or sarcoidosis, delaying accurate diagnosis.
Screen patients for TB if they have a history of persistent cough for more than 2 weeks and a history of possible TB exposure; recent travel in an endemic area; and symptoms of fever, night sweats, unintentional weight loss, shortness of breath, hemoptysis, or chest pain. 1,5 If the primary lesion is large, bacteria can infiltrate the pleural space, causing pleural effusion. Physical examination findings include dullness to percussion and decreased breath sounds in the affected area. 6 Finger clubbing, a late sign, is associated with poor oxygenation. Wasting (loss of body fat and lean tissue) is the result of the inflammatory and immune response. 6 Radiologic abnormalities include hilar or paratracheal adenopathy and cavitary or upper lobe infiltrates. 1,5
Disseminated disease results in lower lobe or miliary pattern infiltrates. Hematologic studies might show anemia and leukocytosis. Extrapulmonary disease can affect any organ in the body including bones, joints, and the genitourinary system, so maintain a high index of suspicion. Rapidly fatal forms include tuberculous meningitis, miliary TB, and lymphatic TB. 6
This asymptomatic and nontransmittable infection is a consequence of exposure to droplet nuclei. Isolating bacteria in culture is not possible. Infection with latent TB is easily recognized as a positive test representing a delayed hypersensitivity response. This response indicates the host's ability to form granulomas around the site of infection containing the organism in a dormant, viable form. These patients are at risk of developing active TB in times of immunosuppression. 8 Viable bacteria have been recovered from TB lesions discovered postmortem in patients who die from other causes. 1 Because of the threat of progression to active disease with potentially drug-resistant strains, latent TB must be identified and treated. 1
The first step in diagnosing TB is clinical suspicion. The distinction between active TB and latent infection is crucial from a clinical and epidemiologic point of view as management is dissimilar. Evaluate HIV status for all patients presenting with probable TB; patients with latent TB who become immunocompromised due to HIV can develop active TB, and coinfection with HIV accounts for the recent increase in TB worldwide. 5 Other comorbidities that can result in progression to active disease include uncontrolled diabetes, sepsis, renal failure, malnutrition, smoking, chemotherapy, organ transplant, and long-term corticosteroid therapy. 3,4,9,11 Clinicians must identify these risk factors for progression in patients with latent TB.
No gold standard exists for confirming latent TB. Infection is suggested by a positive response to intradermal injection of a purified protein derivative of tuberculin, which contains more than 200 antigens found in both Mycobacterium and non-TB Mycobacterium (limiting specificity). Other limitations include low sensitivity in immunocompromised patients, and cross-reactivity with the BCG vaccine. 5 In addition, patients must return in 48 to 72 hours to have the result read. Areas of induration (not redness) are measured in millimeters to determine a positive response ( Table 1 ). The indurated response is consistent with a delayed type IV T-cell-mediated hypersensitivity response. 1

Interferon-gamma release assays, an alternate screening examination, use specific M. tuberculosis antigens not found in the BCG vaccine and nontuberculous Mycobacteria , and should be used for patients who have had the BCG vaccine. 1 They detect the presence of interferon gamma released by sensitized white blood cells after whole blood is incubated with synthetic peptides of strains of mycobacterium, or by the enzyme-linked immunospot technique (requiring isolation of peripheral blood mononuclear cells before incubation). 1 Although these tests have improved specificity, they cannot distinguish latent from active disease. 4,6 Interferon-gamma release assays are recommended by the CDC for identifying TB infection. 1,6
Any patient with a CD4 count less than 200 with atypical pulmonary infiltrates, pleural effusion, and/or lymphadenopathy should undergo TB screening. If the patient's CD4 count is less than 75, pulmonary findings consistent with TB might not be apparent. 4 Consider the possibility of disseminated extrapulmonary TB if the patient has advanced HIV infection. 11
The definitive diagnosis of TB requires culture of acid-fast bacteria Mycobacterium from respiratory secretions with sensitivity testing. 12 Flexible bronchoscopy with bronchial washings is recommended when expectorated sputum samples are inadequate, or produce negative results. 13 Sputum culture is inexpensive with high specificity. The downfall is that the sample concentration of the bacteria can vary depending on timing of collection. Mycobacterium bacilli grow very slowly, with up to 6 weeks needed for detectable growth. 6 The sensitivity of the sputum smear for detection by microscopic examination is 32% to 97%, further emphasizing the need for culture identification. 1,12
Nucleic acid (DNA/RNA) tests such as the polymerase chain reaction (PCR) assay can help diagnose TB. The PCR assay detects small quantities of DNA via amplification methods, and results are available within as little as 2 hours, which can help with early treatment initiation. 1,4,6,13 Limitations include high cost, low sensitivity, and low availability worldwide. 6,13 A new molecular diagnostic test called Xpert MTB/RIF assay detects the M. tuberculosis complex within 2 hours, and has a sensitivity higher than smear microscopy. 4 This test is not approved in the United States but also can identify drug resistance to isoniazid and rifampin, an advantage in countries with high rates of extremely drug-resistant TB. 4
Screening and treatment of latent TB is a cornerstone in the strategy to eliminate TB in the United States. 9 Because patients with latent TB are at risk for progression to active TB, a positive skin test warrants a preventive 9-month regimen of isoniazid, or longer therapy for patients who are immunosuppressed or live in areas of high TB prevalence. 4 Preventive therapy is recommended for patients who are HIV-positive (including pregnant patients); and those in close contact with patients with active TB, infants, and young children. 14 Monotherapy is usually sufficient as bacillary load is low, and resistant mutants are not likely; however, complications with patient adherence to a long regimen are common. 8 Other adverse reactions to isoniazid include drug-related hepatitis resulting in hepatotoxicity and therapy discontinuation.
An effective alternative to daily administration of isoniazid for 9 months is once-weekly directly observed 12 doses of isoniazid and rifapentine. This regimen should not be used in children under age 12 years, patients with HIV on antiretroviral therapy, pregnant women, or women expecting to become pregnant (because of the additional risk for hepatotoxicity). 15 The regimen is recommended for patients who have had recent contact with patients with infectious TB, and for those with positive screening results. 15 This regimen increases compliance up to 90%. 16 A 4-month regimen of rifampin can be considered for patients who cannot take isoniazid and patients exposed to isoniazid-resistant strains. 15 Rifampin is associated with hepatotoxicity and contraindicated in patients on antiretroviral therapy.
Monitor the patient's aminotransferase levels, and evaluate the patient monthly for signs of hepatitis. Asymptomatic elevations in liver enzyme concentrations can occur in 10% to 20% of patients receiving isoniazid. 15 Discontinue treatment if aminotransferase levels exceed three times the upper limit in symptomatic patients, or five times the upper limit in asymptomatic patients. 15
Peripheral neuropathy can occur in 0.2% of patients on isoniazid therapy; the risk is greater in patients who have diabetes, HIV, renal failure, abuse alcohol, or are pregnant. 15 Daily pyridoxine supplementation is recommended for these patients. 15
Treatment of active TB is imperative to reducing the prevalence and incidence of TB. 17 The goal of therapy is to reduce mortality and the emergence of drug-resistant strains. 5,16 Treatment effectiveness depends on early identification, awareness of resistant strains, the patient's HIV status, and adherence to a tolerable regimen. 4 Practices that include monotherapy to treat active disease, or addition of a single agent to failing regimens can result in growth of mutations and resistance. 4
The initial empiric treatment regimen for drug-susceptible TB consists of two phases, with a cure rate of 95% for direct observation therapy. 4 The initial four-drug regimen: isoniazid, rifampin, pyrazinamide, and ethambutol (or streptomycin) is administered over 2 months ( Table 2 ). 14 This intensive phase is designed to kill active and dormant bacteria. Monitor patients weekly for culture conversion, which takes 2 weeks to 3 months in 80% to 90% of patients. 5,14 Patients on pyrazinamide need baseline and periodic serum uric acid assessment and assessment of hepatic function. Elevations in uric acid can lead to hyperuricemia, arthralgias, and gout. Treat elevated levels of uric acid only if the patient is symptomatic. 5 Patients on ethambutol need baseline and periodic tests for visual acuity, blurred vision, reduced red-green color discrimination, and optic neuritis. 5 These adverse reactions are dose-related and reversible; discontinue the medication if the patient develops these symptoms. 5,14 Avoid using these medications in young children who cannot participate in visual screening examinations.

If the organism is resistant to isoniazid, continue rifampin, pyrazinamide, and ethambutol for 6 months. If the organism is susceptible, follow this therapy with the continuation phase: Discontinue pyrazinamide and ethambutol and continue isoniazid and rifampicin for 4 months, unless cultures remain positive. 4,14 This phase reduces the likelihood of the development of drug-resistant mutations (because fewer drugs are needed), eliminates residual bacteria, and prevents reinfection. 5 The regimen is effective for pulmonary and extrapulmonary TB, regardless of the patient's HIV status. 4 Drug-drug interactions can occur between HIV and TB medications, resulting in intolerance, loss of efficacy, toxicity, and treatment disruptions. 4
Although isoniazid, rifampin, and ethambutol are pregnancy category C, they are considered nonteratogenic in pregnancy. 5 Advise patients of the risks and benefits of the medication before treatment. Pyrazinamide may be used in pregnant patients with suspected multidrug-resistant TB, or if alternatives are not available or are less effective. 5
Anti-TB regimens that are comprehensive, effective, and tolerable with fewer drug interactions are urgently needed. Significant effort is being invested into the development of regimens for drug-susceptible TB. Trials are in progress to add fluoroquinolones, or increased doses of rifamycins to shorten regimens to 4 months rather than the current 6. 4
Multidrug-resistant tuberculosis
Multidrug resistance is a challenge to controlling TB worldwide because of inadequate treatment and detection. 3 Multidrug-resistant TB is resistant to at least rifampicin and isoniazid. 18 Isoniazid is known for its strong antibactericidal activity and rifampin for action against dormant bacteria not actively replicating. 14 Multidrug-resistant TB treatment regimens are recommended if the patient has not responded to current treatments, has recurrent TB, has confirmed rifampicin-resistant TB, or has contact with patients with multidrug-resistant TB. 4 The choice of agents is determined by geographic patterns of resistance, previous regimens, underlying medical conditions, and adverse reactions. 4 Empiric treatment should begin after sputum samples are retrieved and without waiting for susceptibility information. The initial intensive phase is 8 months of treatment with at least four second-line drugs daily under direct observation therapy. 4 The continuation phase lasts 20 months if the patient has no history of previous treatment for multidrug-resistant TB, and up to 28 months for patients with recurrent TB, with direct observation therapy to monitor for adherence. 4
Second-line drugs have weak bacteriostatic activity, are less well tolerated, less effective, and have increased toxicity over standard regimens ( Table 3 ). 4,18 Never add a single medication to a failing regimen. 14 Bedaquiline fumarate was recently approved by the FDA for combination therapy for adults with multidrug-resistant TB when an effective regimen is not available. 14,19 The drug's effectiveness and safety profile is unclear. 19 Other drugs are in phase II and III clinical trials. 4,18 Linezolid, clofazimine, and moxifloxacin are in the pipeline for possible treatment of multidrug-resistant TB. 18

Surgical resection is an option and may reduce bacillary burden in patients with multidrug-resistant TB who do not respond to therapy. 14
Extremely drug-resistant TB
This form of TB is resistant to the first-line drugs isoniazid and rifampin, along with the most effective second-line drugs (including at least one fluoroquinolone and one injectable medication). 14 Treatment is limited and requires third-line drugs with greater adverse reactions, especially in patients coinfected with HIV. 4,18 Drug resistance beyond extremely drug-resistant TB is known as total drug-resistant TB, and points out the need for new drug regimens.
All clinicians must take infection control measures to limit TB transmission and outbreaks. Patients with suspected active TB should be admitted to a negative-pressure ventilation isolation room until the diagnosis is definitive. 20 All healthcare providers should receive annual respirator training and fit testing for N-95/high-efficiency particulate respirator masks. 6,20 Healthcare providers should have their TB status screened annually. 20 Patients should wear a mask on leaving the isolation room and all nonurgent procedures should be delayed until patients are noninfectious. Limit visitors, and discourage children from visiting to limit transmission.
TB is a major public health concern worldwide, and remains the world's second most common cause of death from infectious disease after HIV/AIDS. 5 With the emergence of drug-resistant strains and the increased incidence of HIV, clinicians must understand the pathology, clinical manifestations, diagnosis, and management of this disease. Early identification and treatment reduce transmission and prevent increases in patient morbidity and mortality.
tuberculosis; drug-sensitive; drug-resistant; PPD; HIV; immunosuppression
- + Favorites
- View in Gallery
Readers Of this Article Also Read
Lgbtq-competent obesity prevention and management, a review of merkel cell carcinoma, what is causing this patient's shortness of breath, cme post-test, a clinical review of obstructive sleep apnea.
- - Google Chrome
Intended for healthcare professionals
- My email alerts
- BMA member login
- Username * Password * Forgot your log in details? Need to activate BMA Member Log In Log in via OpenAthens Log in via your institution

Search form
- Advanced search
- Search responses
- Search blogs
- Current and future...
Current and future treatments for tuberculosis
- Related content
- Peer review
- Anthony Lee , medical student 1 2 ,
- Yingda Linda Xie , assistant professor 2 3 ,
- Clifton E Barry , senior investigator 2 ,
- Ray Y Chen , associate research clinical 2
- 1 Medical Research Scholars Program, National Institutes of Health, Bethesda, MD, USA
- 2 Tuberculosis Research Section, Laboratory of Clinical Immunology and Microbiology, Division of Intramural Medicine, National Institute of Allergy and Infectious Diseases, National Institutes of Health, Bethesda, MD, USA
- 3 Division of Infectious Diseases, Department of Medicine, Rutgers New Jersey Medical School, Newark, NJ, USA
- Correspondence to RY Chen ray.chen{at}nih.gov
Guidelines on the treatment of tuberculosis (TB) have essentially remained the same for the past 35 years, but are now starting to change. Ongoing clinical trials will hopefully transform the landscape for treatment of drug sensitive TB, drug resistant TB, and latent TB infection. Multiple trials are evaluating novel agents, repurposed agents, adjunctive host directed therapies, and novel treatment strategies that will increase the probability of success of future clinical trials. Guidelines for HIV-TB co-infection treatment continue to be updated and drug resistance testing has been revolutionized in recent years with the shift from phenotypic to genotypic testing and the concomitant increased speed of results. These coming changes are long overdue and are sorely needed to address the vast disparities in global TB incidence rates. TB is currently the leading cause of death globally from a single infectious agent, but the work of many researchers and the contributions of many patients in clinical trials will reduce the substantial global morbidity and mortality of the disease.
Series explanation: State of the Art Reviews are commissioned on the basis of their relevance to academics and specialists in the US and internationally. For this reason they are written predominantly by US authors
Acknowledgment: This research was supported by the Intramural Research Program of the US National Institutes of Health, National Institute of Allergy and Infectious Diseases.
Contributorship: YLX, CEB, and RYC developed the idea for this review. AL, YLX, and RYC performed the literature search. AL, YLX, CEB, and RYC wrote the review. RYC is the guarantor. The corresponding author attests that all listed authors meet authorship criteria and that no others meeting the criteria have been omitted.
Competing interests: The BMJ has judged that there are no disqualifying financial ties to commercial companies. The author declares the following other interests: none.
Further details of The BMJ policy on financial interests are here: https://www.bmj.com/about-bmj/resources-authors/forms-policies-and-checklists/declaration-competing-interests
Provenance and peer review: commissioned; externally peer reviewed.
Log in using your username and password
BMA Member Log In
If you have a subscription to The BMJ, log in:
- Need to activate
- Log in via institution
- Log in via OpenAthens
Log in through your institution
Subscribe from £184 *.
Subscribe and get access to all BMJ articles, and much more.
* For online subscription
Access this article for 1 day for: £50 / $60/ €56 ( excludes VAT )
You can download a PDF version for your personal record.
Buy this article
Click through the PLOS taxonomy to find articles in your field.
For more information about PLOS Subject Areas, click here .
Loading metrics
Open Access
Peer-reviewed
Research Article
Active pulmonary tuberculosis and coronavirus disease 2019: A systematic review and meta-analysis
Roles Conceptualization, Data curation, Formal analysis, Methodology, Project administration, Supervision, Validation, Visualization, Writing – original draft, Writing – review & editing
* E-mail: [email protected]
Affiliation Department of Pulmonary Medicine, Postgraduate Institute of Medical Education and Research, Chandigarh, India

Roles Data curation, Formal analysis, Methodology, Supervision, Validation, Visualization, Writing – original draft, Writing – review & editing
Roles Formal analysis, Methodology, Validation, Visualization, Writing – original draft, Writing – review & editing
- Ashutosh Nath Aggarwal,
- Ritesh Agarwal,
- Sahajal Dhooria,
- Kuruswamy Thurai Prasad,
- Inderpaul Singh Sehgal,
- Valliappan Muthu
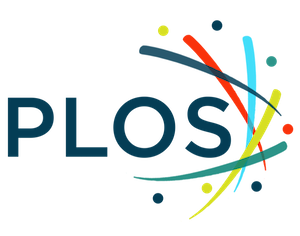
- Published: October 21, 2021
- https://doi.org/10.1371/journal.pone.0259006
- Peer Review
- Reader Comments
The proportion of COVID-19 patients having active pulmonary tuberculosis, and its impact on COVID-19 related patient outcomes, is not clear. We conducted this systematic review to evaluate the proportion of patients with active pulmonary tuberculosis among COVID-19 patients, and to assess if comorbid pulmonary tuberculosis worsens clinical outcomes in these patients.
We queried the PubMed and Embase databases for studies providing data on (a) proportion of COVID-19 patients with active pulmonary tuberculosis or (b) severe disease, hospitalization, or mortality among COVID-19 patients with and without active pulmonary tuberculosis. We calculated the proportion of tuberculosis patients, and the relative risk (RR) for each reported outcome of interest. We used random-effects models to summarize our data.
We retrieved 3,375 citations, and included 43 studies, in our review. The pooled estimate for proportion of active pulmonary tuberculosis was 1.07% (95% CI 0.81%-1.36%). COVID-19 patients with tuberculosis had a higher risk of mortality (summary RR 1.93, 95% CI 1.56–2.39, from 17 studies) and for severe COVID-19 disease (summary RR 1.46, 95% CI 1.05–2.02, from 20 studies), but not for hospitalization (summary RR 1.86, 95% CI 0.91–3.81, from four studies), as compared to COVID-19 patients without tuberculosis.
Active pulmonary tuberculosis is relatively common among COVID-19 patients and increases the risk of severe COVID-19 and COVID-19-related mortality.
Citation: Aggarwal AN, Agarwal R, Dhooria S, Prasad KT, Sehgal IS, Muthu V (2021) Active pulmonary tuberculosis and coronavirus disease 2019: A systematic review and meta-analysis. PLoS ONE 16(10): e0259006. https://doi.org/10.1371/journal.pone.0259006
Editor: Girish Chandra Bhatt, All India Institute of Medical Science - Bhopal, INDIA
Received: July 19, 2021; Accepted: October 8, 2021; Published: October 21, 2021
Copyright: © 2021 Aggarwal et al. This is an open access article distributed under the terms of the Creative Commons Attribution License , which permits unrestricted use, distribution, and reproduction in any medium, provided the original author and source are credited.
Data Availability: All relevant data are within the paper and its Supporting Information files.
Funding: The authors received no specific funding for this work.
Competing interests: The authors have declared that no competing interests exist.
Introduction
The ongoing coronavirus disease 2019 (COVID-19) pandemic is spreading relentlessly, and has affected more than 230 million people worldwide. COVID-19 is associated with worse outcomes in the elderly population, and those with comorbid health conditions such as obesity, diabetes mellitus, hypertension, and cardiovascular disorders [ 1 – 9 ].
Tuberculosis is a destructive pulmonary disease and therefore widely perceived to be associated with increased susceptibility to acquiring COVID-19, and poorer prognosis in patients having both diseases concurrently, especially among people living with human immunodeficiency virus infection (PLHIV). A note from World Health organization (WHO) also anticipated poorer outcomes in these patients [ 10 ]. However, the actual impact of tuberculosis on occurrence and clinical outcomes of COVID-19 is not clear. A case series from Italy reported a benign clinical course for patients having both infections [ 11 ]. An early meta-analysis of six Chinese studies found no association between tuberculosis and COVID‐19 severity or mortality [ 12 ]. Population based data from South Korea also did not suggest tuberculosis to be significantly associated with COVID-19-related mortality [ 13 ]. However, other investigators describe a disproportionately higher rate of adverse clinical outcomes among patients with tuberculosis and COVID-19 [ 14 – 16 ]. Tuberculosis was identified as the commonest comorbidity on verbal autopsy among 70 COVID-19 deaths, and in 10% of whole-body autopsies, in Zambia [ 17 , 18 ]. Two meta-analyses suggest higher odds of underlying tuberculosis among patients with severe COVID-19 and those dying from COVID-19 [ 19 , 20 ]. Due to these inconsistencies, we felt a need to perform a detailed analysis of the available evidence till date. Herein, we evaluate the frequency of concurrent active pulmonary tuberculosis among COVID-19 patients. We also assess if comorbid pulmonary tuberculosis increases the risk of severe disease, hospitalization, or mortality in COVID-19 patients.
We registered our systematic review protocol with the PROSPERO database (registration number CRD42021245835). We followed the Preferred Reporting Items for Systematic Reviews and Meta-Analyses (PRISMA) and the Meta-analysis of Observational Studies in Epidemiology (MOOSE) recommendations for reporting our review [ 21 , 22 ]. An approval from our Institutional Review Board was not necessary as we extracted only summary information from previously published articles.
Search strategy
We initially looked up the PubMed and EMBASE databases for publications indexed till March 31, 2021, and further updated our search on June 30, 2021. We queried the PubMed database using the following search string: (Tuberculosis OR Tubercular OR Tuberculous OR TB OR Mycobacterium OR Mycobacterial) AND (COVID-19 OR “COVID 19” OR COVID19 OR nCoV OR 2019nCoV OR 2019-nCoV OR CoV-2 OR “CoV 2” OR SARS-CoV-2 OR SARSCoV2). The Embase database was similarly searched. We further scanned the WHO compendium of tuberculosis/COVID-19 studies for any additional published studies [ 23 ]. We also examined the bibliographies of selected articles and recent reviews.
Selection of studies
After removing duplicate citations, two reviewers (ANA and RA) screened all the titles and abstracts. We omitted publications not reporting on COVID-19 or tuberculosis. We also excluded experimental, radiological or autopsy studies, case reports, letters to editor not describing original observations, reviews, guidelines, conference abstracts, editorials, and study protocols. Full texts of citations considered potentially suitable by either reviewer were assessed further.
We included a publication for data synthesis if it (a) included patients with COVID-19 confirmed by detection of novel severe acute respiratory syndrome coronavirus 2 (SARS-CoV-2) RNA in respiratory specimens, or strongly suspected on clinical or radiological assessment if a confirmatory test was not available, (b) either described the frequency of patients having concurrent active pulmonary tuberculosis among COVID-19 patients, or reported on any of the following outcomes in COVID-19 patients with and without tuberculosis—severe COVID-19, hospital admission, or mortality. Severe COVID-19 was defined based on institutional or national guidelines, or as per the prevalent guidance from international professional bodies or the World Health Organization. If the same (or substantially overlapping) patient cohort was reported in two or more publications, we included the one describing the largest patient population. In case of any disagreement, consensus between the two reviewers determined study inclusion.
Data extraction and study quality
We obtained information on study design, location and healthcare setting, participant inclusion and exclusion criteria, period of patient enrollment, the source of patient information, and the outcomes reported, from all eligible studies. We used the Newcastle-Ottawa Scale (NOS) to assess methodological quality of studies [ 24 ]. We considered a study to be of good quality if its NOS score was seven or more (out of a maximum possible score of nine).
Statistical analysis
We estimated the percentage of active tuberculosis patients among those with COVID-19 disease in each study and calculated the corresponding 95% confidence interval (95% CI) by Clopper-Pearson exact method [ 25 ]. We also computed the relative risk (RR), and the corresponding 95% CI, for each predefined outcome from each study [ 26 ]. We employed a continuity correction of 0.5 for studies having ‘zero’ cell frequencies prior to these calculations.
We pooled our data using the DerSimonian-Laird random effects model to generate summary estimates [ 27 ]. Freeman-Tukey double arcsine transformation was used to summarize data on proportions [ 28 ]. We assessed between-study heterogeneity through the Higgins’ inconsistency index ( I 2 ), which was considered high for values greater than 0.75 [ 29 ]. The contribution of each study to overall heterogeneity, and its influence on the summary estimate, were assessed through the Baujat’s plot [ 30 ]. For searching the reasons for heterogeneity, we performed subgroup analyses for predefined covariates that included study location and setting, study design, COVID-19 diagnostic standards, description of criteria used to define active tuberculosis, national burden of tuberculosis, and the overall study quality. World Health Organization standards were used to refer to countries as high burden, and to extract country incidence estimates, for tuberculosis [ 31 ]. In a sensitivity analysis, the influence of each study on the summary estimate was also assessed by repeating meta-analysis after iteratively omitting one study at a time. Further, any influential study was identified using a battery of diagnostic tests using Studentized Residuals, Difference in Fits (DFFITS), Cooks Distance, Covariance Ratio, Tau Square, and the contribution of each study in the Q, H2 test statistics value and the weights assigned to these studies [ 32 ]. Publication bias was assessed through Eggers’ test and by visualizing contour-enhanced trim-and-fill funnel plots [ 33 , 34 ]. We utilized the statistical softwares Stata (Intercooled edition 12.0, Stata Corp, USA) and R (version 4.1.1, R Foundation for Statistical Computing, Austria) for analyzing our data.
We identified 3,375 publications from our literature search ( Fig 1 ). We finally selected 43 studies, describing 236,863 patients with COVID-19, for data synthesis [ 35 – 77 ]. Thirty-three (76.7%) of them provided information on one or more of the adverse clinical outcomes of interest ( Table 1 ). There were 30 (69.8%) publications from Asia, and 10 (23.3%) from Africa, with maximum contribution from China (22 studies) ( Table 1 ). One (2.3%) study analyzed data from multiple countries [ 43 ]. All studies evaluated data from retrospective patient cohorts, except for four (9.3%) that collected the information prospectively [ 39 , 40 , 56 , 69 ]. Six (14.0%) studies reported population-based data [ 35 , 36 , 42 , 52 , 59 , 62 ], while the others were conducted in a hospital setting. Two (4.7%) studies also included COVID-19 patients based on high clinical or radiological suspicion [ 39 , 44 ]. All others only studied patients with disease confirmed by the detection of SARS-CoV2 RNA in respiratory specimens. One (2.3%) study did not specify the inclusion criteria [ 46 ]. Only two (4.7%) studies specifically evaluated children [ 63 , 71 ]; others included only adults or described a mixed population. Patient information was retrieved mainly from medical records at participating healthcare facilities, or from surveillance registries or insurance databases ( Table 1 ). Most investigators reviewed patient records or used tuberculosis-related diagnostic codes in databases to identify patients having active tuberculosis ( Table 1 ). Fourteen (32.6%) studies reported human immunodeficiency virus (HIV) seroprevalence in their patient cohorts [ 36 , 37 , 42 , 48 – 50 , 58 – 60 , 62 , 64 , 67 , 69 , 71 ]. Of these, a single study from South Africa provided tuberculosis prevalence and outcome data based on HIV status [ 36 ]. Only six (14.0%) studies were considered high quality ( S1 Table ) [ 36 , 42 , 43 , 52 , 54 , 58 ].
- PPT PowerPoint slide
- PNG larger image
- TIFF original image
https://doi.org/10.1371/journal.pone.0259006.g001
https://doi.org/10.1371/journal.pone.0259006.t001
Proportion of patients with active tuberculosis
The proportion of patients having active pulmonary tuberculosis among COVID-19 patients could be computed from all 43 studies. It ranged from 0.18% to 14.42% ( Fig 2 ). The highest occurrence was noted in a study conducted in a high HIV prevalence South African setting [ 60 ]. All other studies described figures below 6%. Almost all studies reported proportion estimates of comorbid pulmonary tuberculosis among COVID-19 patients that were higher than their corresponding WHO country estimates for annual tuberculosis incidence ( Fig 2 ). The pooled proportion estimate from all 43 studies was 1.07% (95% CI 0.81%-1.36%).
Individual proportion estimates are depicted by solid squares, and the corresponding country estimate of annual tuberculosis incidence by hollow circles.
https://doi.org/10.1371/journal.pone.0259006.g002
There was substantial heterogeneity between the studies ( I 2 94.7%). Baujat’s plot suggested that three studies unduly influenced heterogeneity as well as pooled estimates ( S1 Fig ) [ 36 , 59 , 62 ]. Omitting these three studies from meta-analysis resulted in a slightly higher summary estimate of proportion (1.15%, 95% CI 0.84%-1.50%) with only a minor reduction in heterogeneity ( I 2 86.9%). On influence analysis, a single study with the highest reported proportion of active tuberculosis patients was associated with large values of Studentized residuals, Cook’s distance and DFFITS ( S2 Fig ), and was considered potentially influential [ 60 ]. After removing this study, the pooled proportion estimate from remaining 42 studies was lower at 1.00% (95% CI 0.75%-1.28%) with hardly any reduction in heterogeneity ( I 2 94.5%). On sensitivity analysis, omitting other studies one at a time also did not appreciably influence summary estimates or heterogeneity ( S3 Fig ). On subgroup analysis, studies conducted in low tuberculosis burden or multiple countries showed lesser heterogeneity ( Table 2 ). Overall, the pooled estimates on proportion were much lower from studies conducted in countries not having high tuberculosis burden, as well as from population-based and high-quality studies ( Table 2 ).
https://doi.org/10.1371/journal.pone.0259006.t002
Severe COVID-19
Twenty studies with 24,371 COVID-19 patients, of whom 161 (0.7%) had tuberculosis, provided information on severe COVID-19 [ 35 , 38 , 42 , 44 , 46 , 47 , 49 , 51 , 53 – 58 , 70 , 73 – 77 ]. All, except four (20.0%), of these publications were from China [ 35 , 42 , 49 , 58 ]. Severe COVID-19 was defined based on World Health Organization guidance in three (15.0%) studies [ 35 , 49 , 58 ], recommendations from international professional bodies in two (10.0%) studies [ 44 , 70 ], national guidelines in 11 (55.0%) studies [ 38 , 42 , 46 , 47 , 51 , 53 , 56 , 57 , 73 , 74 , 76 ], and institutional policy in four (20.0%) studies [ 54 , 55 , 75 , 77 ]. Only three (15.0%) studies were considered high quality [ 42 , 54 , 58 ]. All studies, except two, included patients with laboratory confirmed COVID-19 [ 44 , 46 ]. Only one (12.5%) had a prospective study design [ 56 ]. Of the 3431 patients with severe disease in the included cohorts, 36 (1.0%) had underlying tuberculosis. Only four (20.0%) studies reported a RR for severe COVID-19 that significantly exceeded 1.0 ( Fig 3 ) [ 42 , 46 , 56 , 77 ]. COVID-19 patients who also had tuberculosis were 1.46 (95% CI 1.05–2.02) times more likely to develop severe COVID-19 as compared to COVID-19 patients without tuberculosis ( Fig 3 ).
https://doi.org/10.1371/journal.pone.0259006.g003
There was moderate heterogeneity between the studies ( I 2 42.9%). Baujat’s plot indicated that one Korean study unduly influenced heterogeneity as well as pooled estimates [ 42 ]. No additional influential study was identified on formal influence analysis. Omitting this single study from analysis resulted in a lower summary RR estimate (1.35, 95% CI 0.98–1.87) and lesser heterogeneity ( I 2 29.5%). On sensitivity analysis, omitting other studies one at a time did not significantly affect heterogeneity ( S4 Fig ). On subgroup analysis, studies conducted in Africa or in low tuberculosis burden or multiple countries, as well as population-based studies, showed negligible heterogeneity ( Table 3 ). Overall, the pooled RR estimates were much higher from studies conducted in countries not having high tuberculosis burden, as well as from population-based studies ( Table 3 ). There was no significant publication bias ( S5 Fig ).
https://doi.org/10.1371/journal.pone.0259006.t003
Need for hospitalization
Four publications with 28,438 COVID-19 patients, of whom 479 (1.7%) had tuberculosis, provided data on hospitalization due to COVID-19 [ 36 , 45 , 52 , 71 ]. All these studies were from high tuberculosis burden countries (two from South Africa, and one each from China and Philippines), had a retrospective study design, and included patients with laboratory confirmed COVID-19. Two (50.0%) studies were of high quality [ 36 , 52 ]. Overall, 20.6% of patients were hospitalized. Of the 5853 patients who required hospitalization in the included cohorts, 227 (3.9%) had underlying tuberculosis. Two studies reported a RR for hospitalization that statistically significantly exceeded 1.0 ( Fig 3 ) [ 36 , 71 ]. COVID-19 patients who also had tuberculosis were 1.86 (95% CI 0.91–3.81) times more likely require hospitalization as compared to COVID-19 patients without tuberculosis ( Fig 3 ). This pointed to the absence of any statistically significant risk of hospitalization among COVID-19 patients with tuberculosis.
There was considerable heterogeneity between the studies ( I 2 97.5%). A subgroup analysis was not undertaken due to small number of studies. There was no significant publication bias ( S5 Fig ).
Seventeen studies with 42,321 COVID-19 patients, of whom 632 (1.5%) had tuberculosis, reported on deaths due to COVID-19 [ 36 , 39 , 41 – 43 , 45 , 47 – 50 , 52 , 57 , 60 , 63 , 65 – 67 ]. All studies were conducted in high tuberculosis burden countries, except one from South Korea and another that combined data from multiple nations [ 42 , 43 ]. All studies, except one, included patients with laboratory confirmed COVID-19 [ 39 ]. Only one publication had a prospective study design [ 39 ]. Four (23.5%) studies were considered as high quality [ 36 , 42 , 43 , 52 ]. Of the 2822 patients who died in the included cohorts, 97 (3.4%) had underlying tuberculosis. Only four (23.5%) studies reported RR for mortality that clearly exceeded 1.0 ( Fig 3 ) [ 36 , 42 , 45 , 52 ]. The confidence limits for all other studies were wide ( Fig 3 ). COVID-19 patients who also had tuberculosis were 1.93 (95% CI 1.56–2.39) times more likely to die as compared to COVID-19 patients without tuberculosis ( Fig 3 ).
There was only mild heterogeneity between the studies ( I 2 21.5%). Inspection of Baujat’s plot indicated that four studies unduly affected heterogeneity as well as pooled estimates [ 36 , 42 , 52 , 67 ]. Two of these were also considered to be influential on formal influence analysis [ 52 , 67 ]. Omitting these four studies from analysis lowered the summary RR estimate (1.65%, 95% CI 1.25–2.18) and resulted in negligible heterogeneity ( I 2 0.0%). On sensitivity analysis, heterogeneity could also be further decreased by individually omitting three of these studies one at a time ( S4 Fig ) [ 36 , 42 , 67 ]. Stratification by whether criteria for tuberculosis definition were specified in the studies resulted in homogeneity in either group ( Table 3 ). On subgroup analysis, studies conducted outside Asia or Africa or in low tuberculosis burden or multiple countries, population-based studies, those including patients with a clinico-radiological diagnosis of COVID-19, and low-quality publications showed negligible heterogeneity ( Table 3 ). There was no significant publication bias ( S5 Fig ).
We found that 0.99% of the COVID-19 patients had active pulmonary tuberculosis. These patients showed higher risk for mortality, but not for severe disease or hospitalization, than COVID-19 patients without tuberculosis. Our data synthesis summarizes far greater number of studies than previous meta-analyses and provides information both on tuberculosis frequency and COVID-19 outcome estimates [ 12 , 19 , 20 ]. Unlike previous meta-analyses that reported summary odds ratios, we present summary RR estimates for adverse clinical outcomes, which are much easier to interpret and understand in a clinical setting.
The summary proportion of those with active pulmonary tuberculosis among COVID-19 patients appears higher than the recent WHO estimates for annual incidence of tuberculosis in some of the high tuberculosis burden countries where most of the studies were conducted (China 0.06%, India 0.19%, Nigeria 0.22%, Philippines 0.55%, and South Africa 0.61%) [ 31 ]. However, this proportion of active tuberculosis is lower than the generally reported proportion of other comorbid conditions, like diabetes or hypertension [ 5 , 8 ]. Whether the lower tuberculosis proportion is due to under-reporting or under-recognition of active tuberculosis among COVID-19 patients, or to safeguarding strategies commonly employed by people with respiratory disorders, is not certain. However, when patients with active pulmonary tuberculosis do acquire COVID-19, there is a significantly greater risk (about two-fold higher) of COVID-19 mortality. Our summary estimate for relative risk of mortality in COVID-19 patients having tuberculosis is quite similar to the relative risk estimates of mortality for COVID-19 patients having other comorbid conditions (like diabetes, hypertension, or cardiovascular diseases) widely known to adversely affect prognosis in COVID-19 patients [ 1 – 3 ]. It is likely that superadded COVID-19 pneumonia in a lung that is already structurally damaged by tuberculosis may manifest as more severe disease. Importantly, local alterations in lung immunity resulting from active pulmonary tuberculosis can also adversely influence host response to SARS-CoV-2 virus. Recent in-vitro data from COVID-19 patients with active pulmonary tuberculosis has shown an attenuated interferon-gamma response after stimulation of whole blood with peptides derived from SARS-CoV-2 spike protein, in contrast to a normal response to Mycobacterium tuberculosis -specific antigens [ 78 ].
There are several similarities between COVID-19 and pulmonary tuberculosis. In several countries, COVID-19 too is a stigmatizing disorder, much like tuberculosis. Both diseases show airborne transmission when people are in close contact. Both present with similar symptoms like fever and cough. This can complicate decision-making, especially is nations with high tuberculosis burden. Although several countries have proposed bidirectional screening of both COVID-19 and pulmonary tuberculosis patients, such policy remains difficult to implement in resource-constrained settings. This might contribute to underdiagnosis of tuberculosis in COVID-19 patients. As it is, under-reporting of tuberculosis is a problem that is globally recognized. This is further compounded by reduced access to tuberculosis diagnosis and treatment as a result of COVID-19 related restrictions. It is therefore possible that our calculations regarding pulmonary tuberculosis among COVID-19 patients might be an underestimate.
Our systematic review has a few limitations. Due to the dynamic nature of the pandemic, and the lag between data collection and publication of results, most studies provide information from the initial months of 2020 and from regions that were severely afflicted earlier. Thus, the figures may not truly represent the patient data from all the geographic locations. Also, most of the included studies had a retrospective design, and collated data from medical records that were likely completed in an overwhelmed health system. This could have resulted in both underreporting as well as misclassification of comorbid health conditions. Several studies reported only on inpatients who have a higher probability of adverse outcomes compared to patients in the community. Only 15.6% of the included studies were of sufficiently high quality. There were differences in healthcare strategies regarding SARS-CoV-2 testing and admission/transfer criteria, variability in institutional practices in the timing of investigations and other evaluations, and the level and extent of medical intervention available to patients. Such heterogeneity can restrict the generalizability of our results. We cannot rule out an overestimation from lack of adjustment for potential confounders (like age, HIV status, other comorbid health conditions, or other patient characteristics) as we focused on univariate estimates. In particular, only one South African study reported on tuberculosis frequency data and outcome parameters stratified by HIV status, and there is need to gather more information on the impact of HIV on COVID-19 and tuberculosis associations.
In summary, the available evidence suggests that COVID-19 patients show relatively higher proportion of concurrent active pulmonary tuberculosis. Active pulmonary tuberculosis significantly increases the risk of severe COVID-19 and COVID-19-related mortality.
Supporting information
S1 table. details of newcastle-ottawa scale scoring for study quality..
https://doi.org/10.1371/journal.pone.0259006.s001
S1 Fig. Baujat’s plot for studies reporting prevalence of tuberculosis among COVID-19 patients.
https://doi.org/10.1371/journal.pone.0259006.s002
S2 Fig. Influence statistics for studies reporting prevalence of tuberculosis among COVID-19 patients.
https://doi.org/10.1371/journal.pone.0259006.s003
S3 Fig. Sensitivity analysis for studies reporting prevalence of tuberculosis among COVID-19 patients.
https://doi.org/10.1371/journal.pone.0259006.s004
S4 Fig. Sensitivity analysis for studies reporting outcomes of patients COVID-19 patients with comorbid tuberculosis.
https://doi.org/10.1371/journal.pone.0259006.s005
S5 Fig. Contour-enhanced trim-and-fill funnel plots.
https://doi.org/10.1371/journal.pone.0259006.s006
- View Article
- PubMed/NCBI
- Google Scholar
- 23. World Health Organization. Compendium of TB/COVID-19 studies. www.who.int/teams/global-tuberculosis-programme/covid-19/compendium [Accessed July 1, 2021].
- 31. World Health Organization. Global Tuberculosis Report 2020. Geneva: World Health Organization; 2020.
ORIGINAL RESEARCH article
Spatiotemporal analysis of tuberculosis in the hunan province, china, 2014–2022.
- 1 Chinese Center for Disease Control and Prevention, Beijing, China
- 2 Department of Science and Education, Hunan Chest Hospital, Changsha, China
- 3 Department of Tuberculosis Control and Prevention, Hunan Chest Hospital, Changsha, China
- 4 Hunan Chest Hospital, Changsha, China
Background: Pulmonary tuberculosis (PTB) is a major infectious disease that threatens human health. China is a high tuberculosis-burden country and the Hunan Province has a high tuberculosis notification rate. However, no comprehensive analysis has been conducted on the spatiotemporal distribution of PTB in the Hunan Province. Therefore, this study investigated the spatiotemporal distribution of PTB in the Hunan Province to enable targeted control policies for tuberculosis.
Methods: We obtained data about cases of PTB in the Hunan Province notified from January 2014 to December 2022 from the China Information System for Disease Control and Prevention. Time-series analysis was conducted to analyze the trends in PTB case notifications. Spatial autocorrelation analysis was conducted to detect the spatial distribution characteristics of PTB at a county level in Hunan Province. Space-time scan analysis was conducted to confirm specific times and locations of PTB clustering.
Results: A total of 472,826 new cases of PTB were notified in the Hunan Province during the 9-year study period. The mean PTB notification rate showed a gradual, fluctuating downward trend over time. The number of PTB notifications per month showed significant seasonal variation, with an annual peak in notifications in January or March, followed by a fluctuating decline after March, reaching a trough in November or December. Moran’s I index of spatial autocorrelation revealed that the notification rate of PTB by county ranged from 0.117 to 0.317 during the study period, indicating spatial clustering. The hotspot areas of PTB were mainly concentrated in the Xiangxi Autonomous Prefecture, Zhangjiajie City, and Hengyang City. The most likely clustering region was identified in the central-southern part of the province, and a secondary clustering region was identified in the northwest part of the province.
Conclusion: This study identified the temporal trend and spatial distribution pattern of tuberculosis in the Hunan Province. PTB clustered mainly in the central-southern and northwestern regions of the province. Disease control programs should focus on strengthening tuberculosis control in these regions.
1 Introduction
Pulmonary tuberculosis (PTB) is a major infectious disease that seriously threatens human health. According to the WHO Global Tuberculosis Report 2023 ( 1 ), an estimated 10.6 million new cases of PTB occurred globally in 2022, with 1.3 million deaths. PTB remains an important public health problem. After decades of efforts, the incidence of tuberculosis has gradually declined in China. However, the incidence of tuberculosis within the country varies by region, with some areas still experiencing major outbreaks ( 2 , 3 ). The Hunan Province, located in central-southern China, has reported high numbers of cases and PTB notification rates. In 2022, 43,976 new PTB cases were notified, with a notification rate of 66.40/100,000, which was higher than the national average. Therefore, more effective control strategies are urgently needed to curb the spread of tuberculosis in the Hunan Province.
Spatiotemporal analysis methods have been used extensively to investigate the distribution and variation patterns of PTB in recent years. Studies have been conducted in several countries, including Russia ( 4 ), Brazil ( 5 , 6 ), Uganda ( 7 ), and Peru ( 8 ). In China, researchers such as Zhang et al. ( 9 ) have studied the spatiotemporal distribution characteristics of tuberculosis at a provincial level, whereas others such as Liu et al. ( 3 ) have conducted similar analyses at a prefecture level. Studies of the spatiotemporal distribution of tuberculosis have been conducted in Beijing ( 10 ), Zhejiang ( 11 , 12 ), Chongqing ( 13 ), and Qinghai ( 14 ) Provinces. In the Hunan Province, Alene et al. ( 15 ) examined spatiotemporal distribution patterns, and Zheng et al. ( 16 ) investigated the spatial clustering and hotspot areas of smear-positive PTB notifications in the Hunan Province in 2012 and 2013. However, to our knowledge, no comprehensive analysis has been conducted on the spatiotemporal distribution of PTB in the whole of the Hunan Province. The current spatiotemporal distribution and trends in the spatiotemporal distribution of PTB in the Hunan Province remain unclear and warrant further investigation.
This study aimed to analyze the temporal distribution characteristics of tuberculosis in the Hunan Province, examining the clustering of PTB notifications at a county level to identify hotspot and cold-spot areas of incidence. Through spatiotemporal scanning analysis, this study aimed to pinpoint specific locations of tuberculosis incidence clustering and assess the magnitude of disease risk in these clusters. By identifying the key regions of PTB incidence in the Hunan Province, these results provide a source of reference for developing targeted tuberculosis control strategies.
2 Materials and methods
2.1 overview of the study area.
The Hunan Province is in the central-southern region of China ( Figure 1 ), which covers an area of 211,800 square kilometers. Its terrain is characterized by mountains and hills, with mountainous areas covering 51.2%, hills and plateaus covering 29.3%, plains covering 13.1%, and water surfaces covering 6.4% of the total provincial area. The number of permanent residents was 66.04 million in 2022. The Hunan Province includes 14 prefecture-level administrative divisions, with 122 county-level administrative divisions ( 17 ).
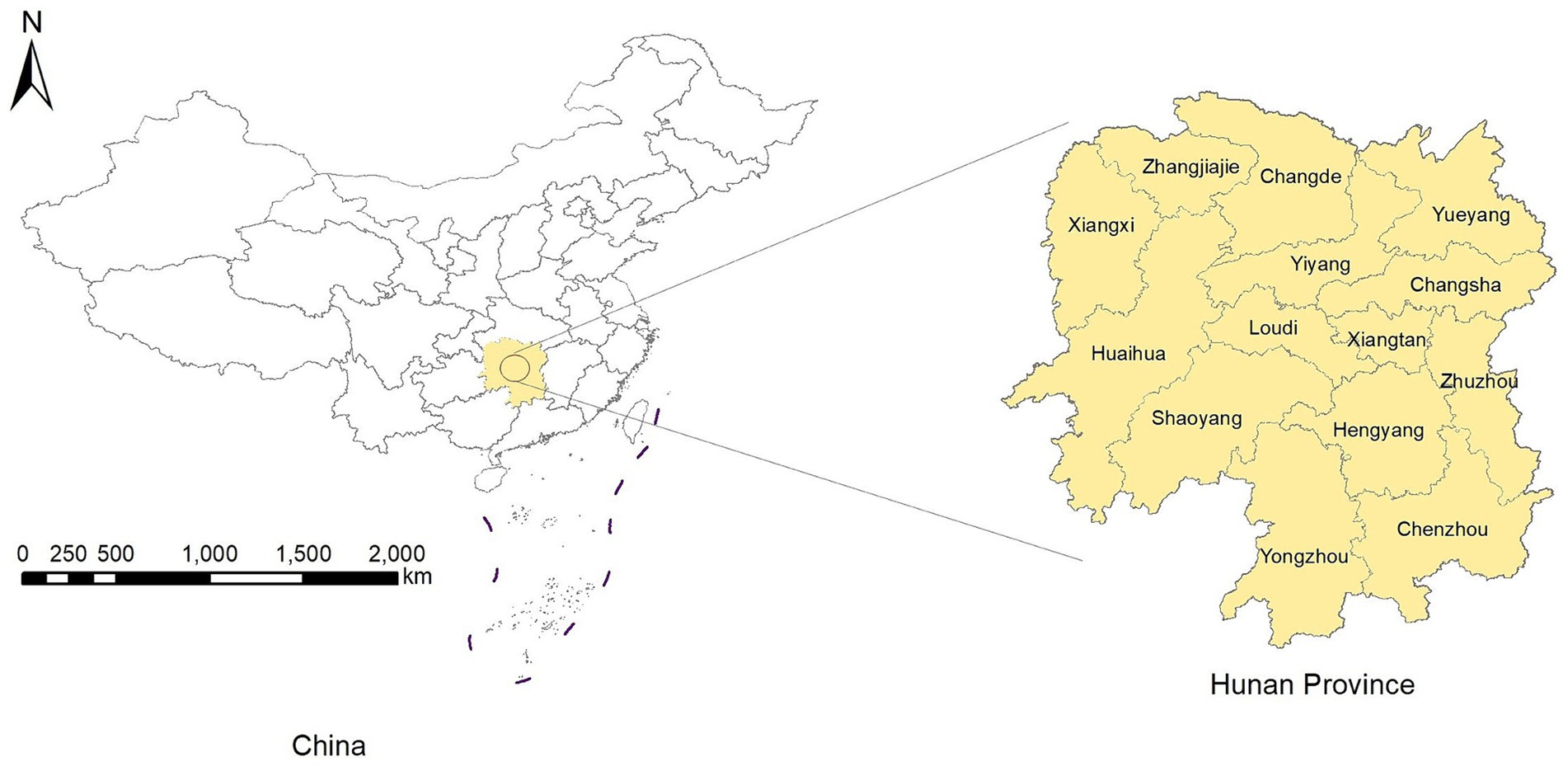
Figure 1 . Location of the Hunan Province.
2.2 Data collection
Data on PTB notifications for each county in the Hunan Province from January 2014 to December 2022 were download from the China Information System for Disease Control and Prevention (CISDCP). The dataset included the number of PTB notifications for each county by month. PTB notifications included both laboratory-confirmed and clinically diagnosed cases. From January 2014 to April 2018, the diagnostic criteria for tuberculosis were based on the National Health Commission of the People’s Republic of China WS 288-2008, and from May 2018 to December 2022, the diagnostic criteria for tuberculosis were based on the National Health Commission of the People’s Republic of China WS 288-2017 ( 18 ). Population data for each county in the Hunan Province from 2014 to 2022 were obtained from the Hunan Statistical Yearbook (2015–2023). To calculate the mean PTB notification rate, we summed the number of cases notified by county for the 9-year period and divided them by the total population of each county for the same period.
The overall PTB notification rate and the notification rate of laboratory-confirmed cases of PTB were used as indicators of the effectiveness of control programs. The overall PTB notification rate included laboratory-confirmed cases, laboratory-negative cases, and cases with no laboratory results. According to the 2019 notification on the adjustment of the classification of infectious disease reports for PTB ( 19 ), laboratory-confirmed cases of PTB include smear-positive cases; smear-negative, culture-positive cases; and cases testing positive on nucleic acid amplification tests (NAATs). Since January 1, 2017, cases of rifampicin-resistant tuberculosis have been recorded in CISDCP ( 20 ). Laboratory-negative PTB refers to patients with PTB whose sputum smears and cultures are both negative, and NAAT results are negative or unavailable. PTB with no laboratory results refers to patients with tuberculosis symptoms or tuberculous pleurisy, but no sputum smear, culture, or NAAT results.
2.3 Statistical analysis
2.3.1 time-series analysis.
In the time-series analysis, we analyzed the number of notified PTB cases in the Hunan Province from January 2014 to December 2022 (108 months). Using time as the horizontal axis and the number of notified PTB cases per month as the vertical axis, we plotted a curve showing the monthly PTB cases in the Hunan Province over time. The tuberculosis incidence was analyzed by observing the trend in the curve. The analyses were conducted using Microsoft Excel 2019 (Microsoft Corporation, Redmond, WA, United States).
2.3.2 Spatial autocorrelation analysis
Spatial autocorrelation analysis was conducted to investigate whether the observed values at a particular location in a spatial area were correlated with similar observed values in neighboring areas. The analysis included global and local spatial autocorrelation. Global spatial autocorrelation can be classified into positive spatial autocorrelation, negative spatial autocorrelation, and spatial randomness (i.e., random distribution) based on the spatial distribution characteristics. Spatial autocorrelation analysis quantifies the type of spatial data correlation, explores clustering, and examines the process through which spatial features change over time, thereby identifying risk factors for disease in the study region. For the global spatial autocorrelation analysis, we used Moran’s I ( 21 ), which is widely applied as a statistical index in spatial epidemiology ( 11 , 13 ). The Moran’s I index can range between −1 and 1. A positive Moran’s I value closer to 1 indicates higher clustering, whereas a negative value closer to −1 suggests a more dispersed distribution. A value of zero indicates a random distribution. We used this method to confirm the spatial distribution characteristics of the PTB notification rate at a county level in Hunan Province from 2014 to 2022. The Z-score was calculated to assess the significance of the Moran’s I estimate. If Moran’s I is greater than 0 and the Z-score is greater than or equal to 1.96, the distribution of disease is assumed to be spatially clustered and statistically aggregated ( 22 ).
Local spatial autocorrelation analysis is used to describe spatial correlation patterns, locate specific clustering areas, and investigate local spatial instability, thus revealing spatial heterogeneity among data. In this study, the local Gi * statistic was calculated to assess local spatial autocorrelation ( 23 ) and to identify hotspot and cold-spot areas of PTB notifications. A significant positive value, such as Gi* ≥ 1.96, indicates that high values in the locality are clustered more than those in other areas. Conversely, a significant negative value, such as Gi* ≤ −1.96, indicates that low values in the locality are clustered less than those in other areas. These analyses were conducted using ArcGIS 10.2 software (ESRI Inc., Redlands, CA, United States).
2.3.3 Space-time scan statistic
The space-time scan statistic was proposed by Kulldorff ( 24 ) in 1995 to observe and infer the spatiotemporal clustering of diseases. It can detect abnormal changes in the number of occurrences of a specific event (disease) within a spatiotemporal range and test whether these changes are due to random variation. That is, it investigates whether disease clustering exists within the study region, the exact location of the clustering, and the magnitude of clustering risk, and tests whether this clustering has statistical significance. Currently, it is widely used in tuberculosis research ( 3 , 11 , 13 ).
In this study, the space-time scan statistic model used a Poisson model and focused on areas with high PTB notification rates. The space-time scan window is a cylinder in which both spatial and temporal dimensions are constantly changing, corresponding to changes in the radius and height of the cylinder base. Simultaneously, the center of the circle at the base of the cylinder moves between the center points of each spatial unit. Each time the center, radius, and height of the cylindrical window change, a log-likelihood ratio (LLR) is calculated to compare the risk inside and outside the window. The space-time scan uses the Poisson model for every position and scale of the space-time scan window. The null hypothesis assumes that the spatial distribution of the disease is completely random, whereas the alternative hypothesis suggests an increased risk of disease occurrence inside the scan window relative to outside the scan window. For each scan window, the LLR test statistic is calculated based on the disease occurrences inside and outside the window to compare the risk. The window with the maximum LLR is considered the most likely cluster, known as the primary cluster, whereas other windows displaying statistically significant LLR values are defined as secondary clusters ( 25 ). These analyses were conducted using SaTScan version 9.5 (Kulldorff, Boston, MA, United States).
2.4 Ethical review
This study was approved by the Medical Ethics Committee of Hunan Chest Hospital. All personal information and privacy protection were carried out in accordance with the ethical requirements. The requirement for informed consent did not apply because the notification data were aggregated, and no individual-level data were analyzed.
3.1 Overview of PTB in the Hunan Province
From 2014 to 2022, 472,826 cases of PTB were notified in the Hunan Province; of them, 205,176 cases were laboratory-confirmed, 243,571 cases were laboratory-negative, and 24,079 cases did not have information available on microbiological status. The percentage of laboratory-confirmed PTB increased from 38.43% in 2014 to 57.74% in 2022, whereas the percentage of cases with negative results decreased from 55.49% in 2014 to 38.56% in 2022 ( Table 1 ). The mean annual PTB notification rate in the Hunan Province between 2014 and 2022 was 77.55/100,000. The mean annual PTB notification rate in the province decreased from 85.88/100,000 in 2014 to 66.40/100,000 in 2022, showing a gradual downward trend with fluctuations. The annual notification rate of laboratory-confirmed PTB increased from 33.00/100,000 in 2014 to 38.34/100,000 in 2022, showing a fluctuating gradual upward trend ( Figure 2 ). In contrast, the notification rate of laboratory-negative PTB decreased from 47.65/100,000 in 2014 to 25.60/100,000 in 2022, showing a downward trend ( Figure 2 ).
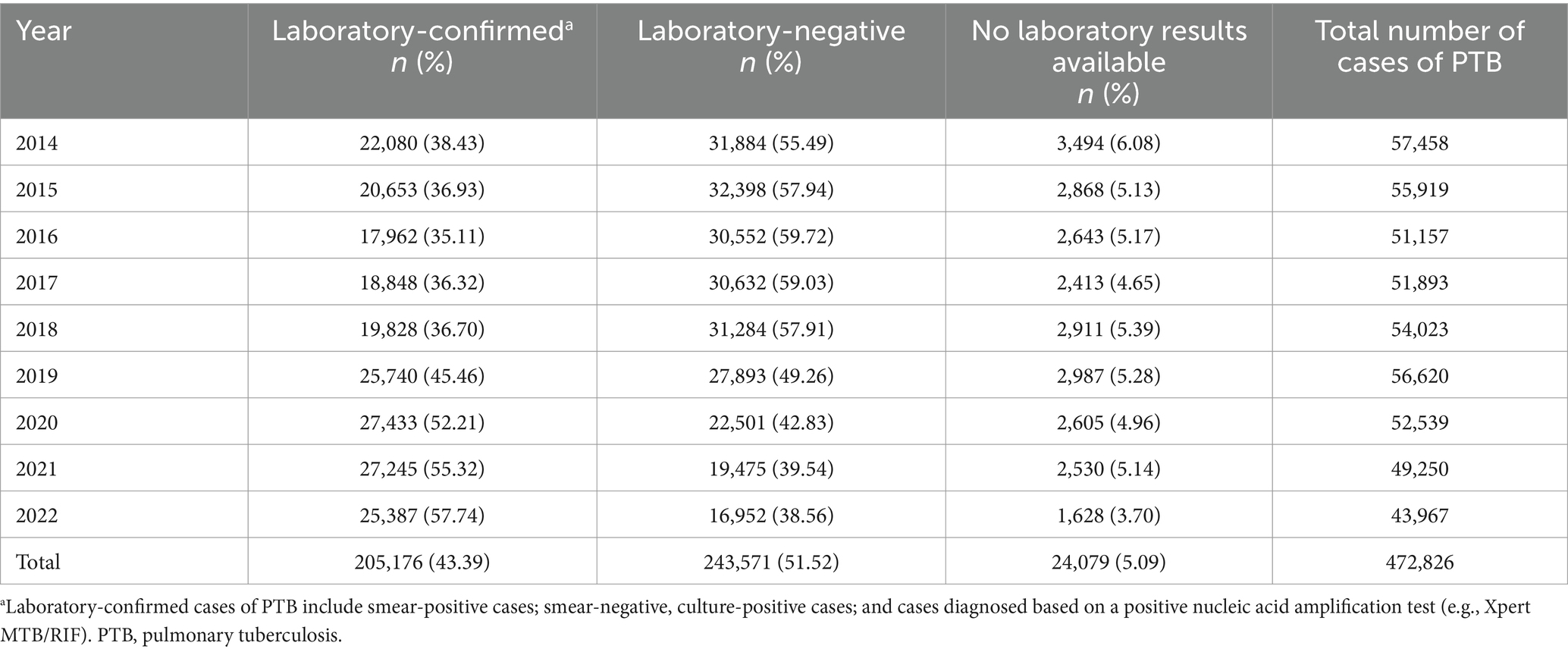
Table 1 . Laboratory confirmation of cases of PTB notified in the Hunan Province, China from 2014 to 2022.
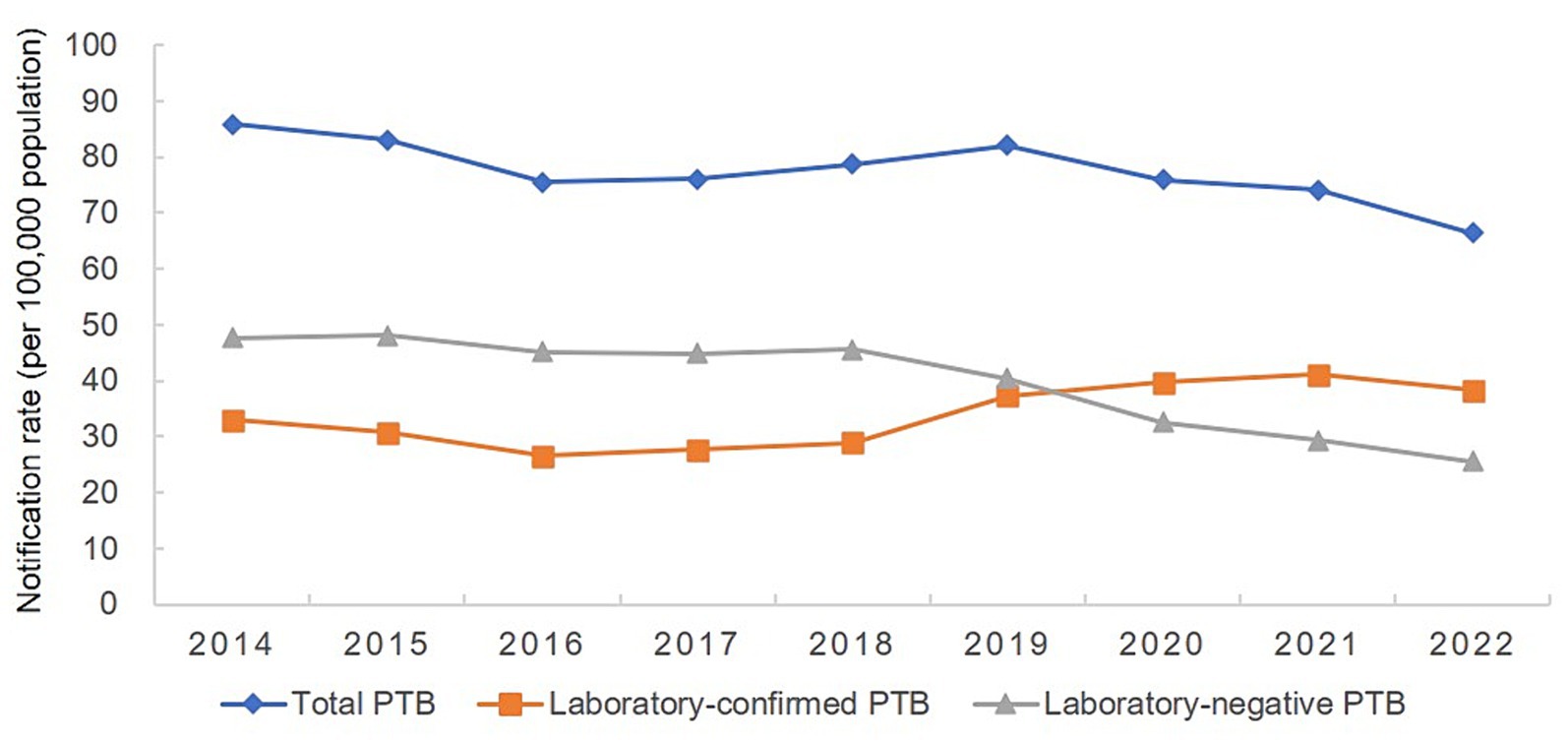
Figure 2 . Annual pulmonary tuberculosis notification rate in the Hunan Province, China from 2014 to 2022.
3.2 Temporal trends in PTB case notifications
The number of PTB notifications per month showed significant seasonal variation from 2014 to 2019 ( Figure 3 ). The number of notifications peaked in January or March every year, followed by a fluctuating decline after March, and reached a trough in November or December. However, this pattern was disrupted from 2020 to 2022, with peaks occurring in June, April, and July, in 2020, 2021, and 2022, respectively, although the troughs still occurred in December each year.
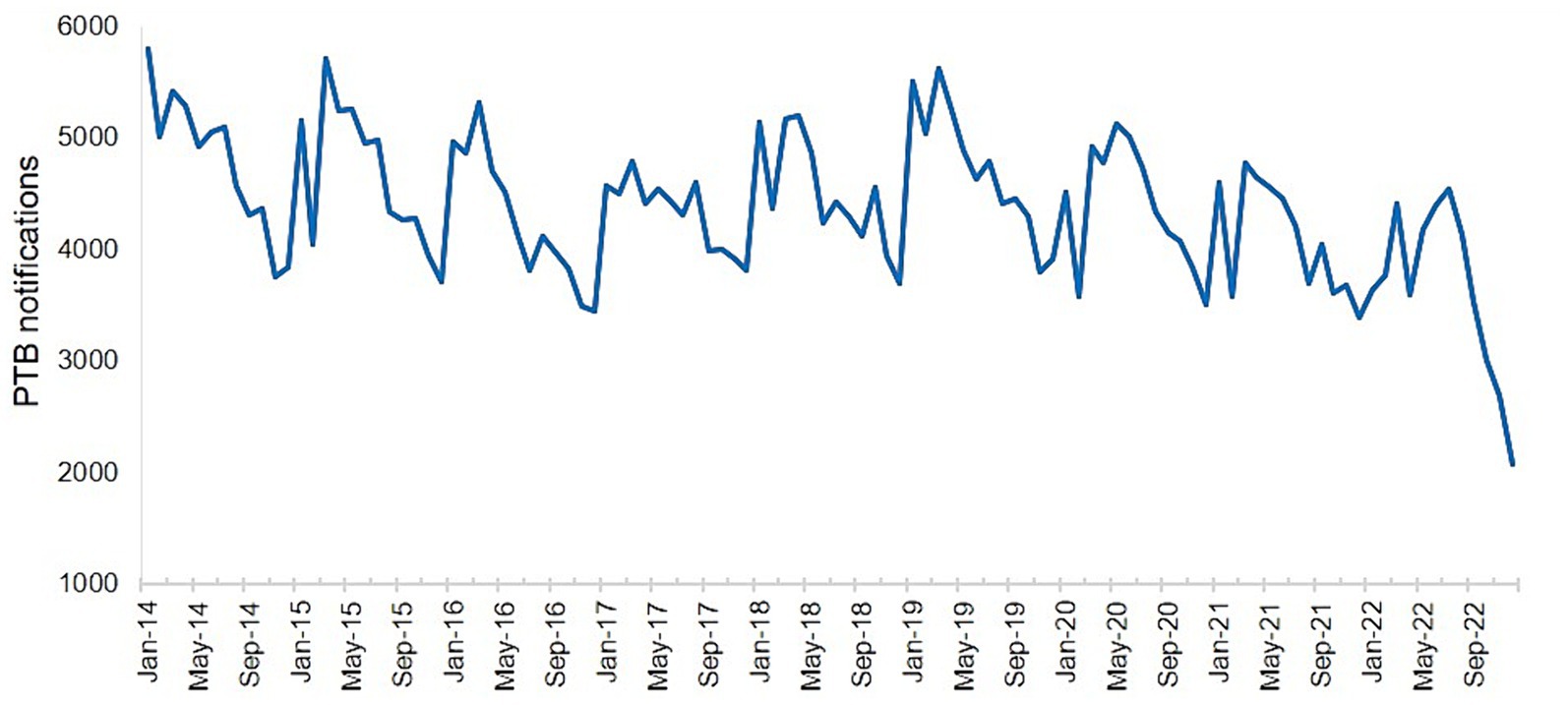
Figure 3 . Number of notified cases of pulmonary tuberculosis in the Hunan Province by month from January 2014 to December 2022.
3.3 Spatial distribution of PTB case notifications
The PTB notification rate in the Hunan Province between 2014 and 2022 varied by county and district ( Figure 4 ). Over the 9-year period, the counties with the highest mean PTB notification rates were the Hengdong, Sangzhi, Cili, Hengshan, and Fenghuang Counties. The global spatial autocorrelation analysis results showed that Moran’s I of the annual PTB notification rate at a county level ranged from 0.117 to 0.317 between 2014 and 2022 ( p < 0.05) ( Table 2 ), indicating that the occurrence of PTB exhibited a positive global spatial autocorrelation and was clustered.
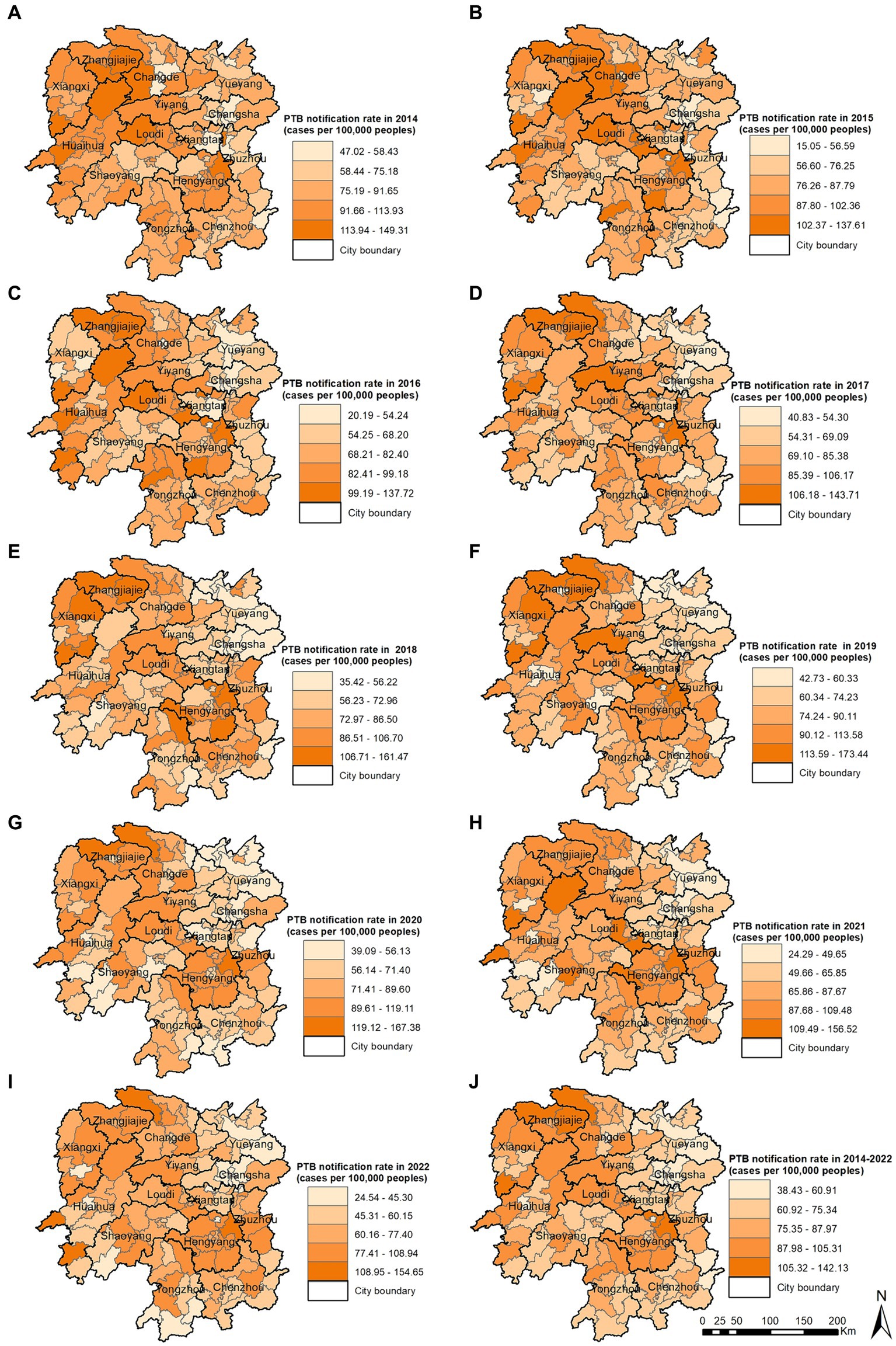
Figure 4 . Pulmonary tuberculosis notification rate in 2014 (A) , 2015 (B) , 2016 (C) , 2017 (D) , 2018 (E) , 2019 (F) , 2020 (G) , 2021 (H) , 2022 (I) , 2014–2022 (J) .
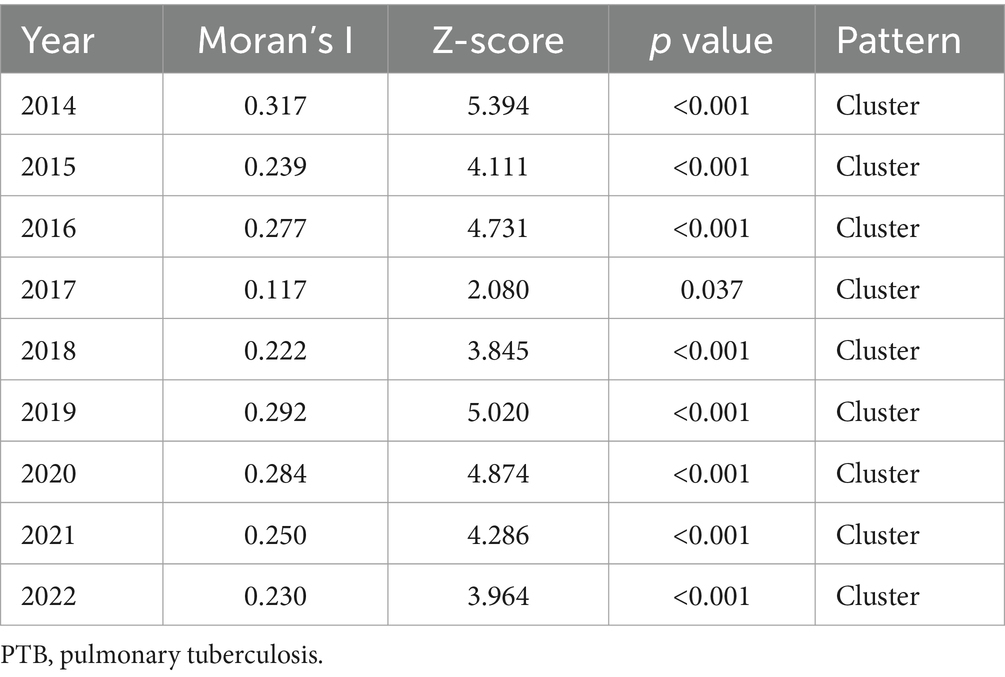
Table 2 . Global spatial autocorrelation analysis of the annual PTB notification rate in the Hunan Province, China from 2014 to 2022.
The local spatial autocorrelation analysis results revealed that the hotspot and cold-spot areas of disease incidence in the Hunan Province changed annually from 2014 to 2022. The hotspot areas were mainly concentrated in the Xiangxi Autonomous Prefecture, Zhangjiajie City, and Hengyang City, and in some adjacent areas such as the Yuanling County in Huaihua City, Shimen County in Changde City, Xinhua County in Loudi City, Anhua County in Yiyang City, Qiyang City in Yongzhou City, and You County in Zhuzhou City. From 2018 to 2022, the hotspot areas in the Xiangxi Autonomous Prefecture gradually decreased annually and disappeared in 2021 and 2022; however, hotspot areas gradually formed in the Hengyang City from 2018 to 2022. The cold-spot areas of disease incidence gradually expanded from the urban zones of Changsha, Zhuzhou, and Xiangtan in 2014 to the entire cities of Changsha, Zhuzhou, and Xiangtan, and some areas of Yueyang, by 2022 ( Figures 5A – I ). Comparison of the mean PTB notification rate and the clustering of laboratory-confirmed cases of PTB over the 9-year study period revealed patterns in hotspot and cold-spot areas. However, the hotspot areas of laboratory-confirmed cases of PTB also included the Jishou City and Fenghuang County in the Xiangxi Autonomous Prefecture, and the Mayang Miao Autonomous County and Chenxi County in Huaihua City ( Figures 5J , K ).
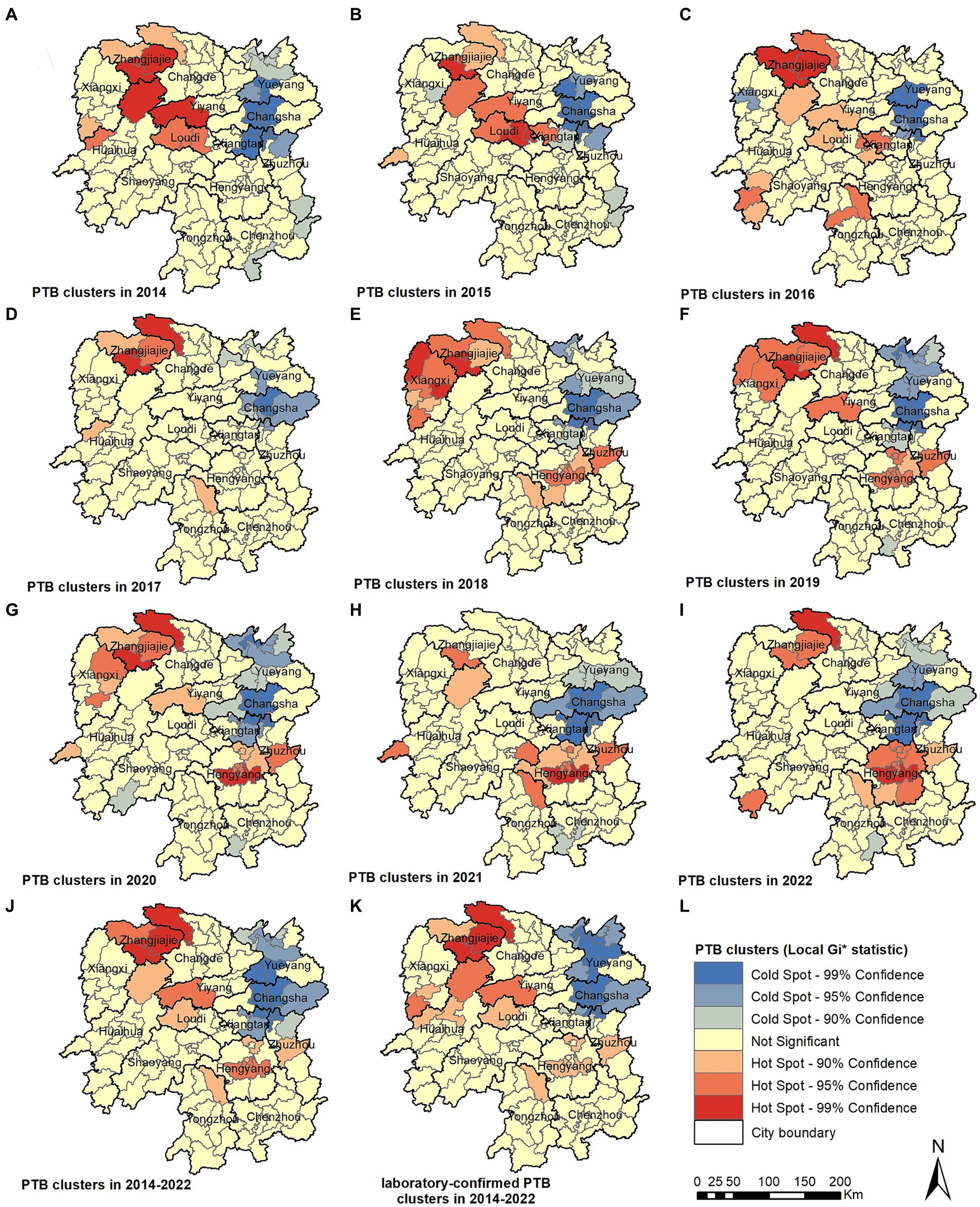
Figure 5 . Pulmonary tuberculosis clusters in 2014 (A) , 2015 (B) , 2016 (C) , 2017 (D) , 2018 (E) , 2018 (F) , 2020 (G) , 2021 (H) , 2022 (I) , 2014–2022 (J) , laboratory-confirmed PTB clusters in 2014–2022 (K) .
3.4 Spatiotemporal clustering analysis using SaTScan
The spatiotemporal scanning analysis results showed two distinct clusters of PTB incidence in the Hunan Province between 2014 and 2022. The most likely cluster was in the central-southern part of the Hunan Province, including the entire area of the Hengyang City, Anren County in Chenzhou City, Chaling County and You County in Zhuzhou City, Shuangfeng County in Loudi City, and Qiyang City in Yongzhou City, comprising 17 counties and districts. The clustering period was from March 1, 2018, to August 31, 2022, during which 47,338 cases of PTB were confirmed. The PTB notification rate within the clustered area was 1.36 times higher than that in areas outside the cluster. The secondary cluster was situated in the northwest region of the Hunan Province, encompassing the entire Xiangxi Autonomous Prefecture, Zhangjiajie City, eight county-level administrative regions in Huaihua City, Taoyuan County and Shimen County in Changde City, Anhua County in Yiyang City, and Xinhua County in Loudi City, comprising 25 counties and districts. The clustering period was from January 1, 2014, to May 31, 2018, during which 50,877 cases of PTB were notified. The PTB notification rate within the clustered area was 1.3 times higher than that in areas outside the cluster ( Table 3 ; Figure 6 ).
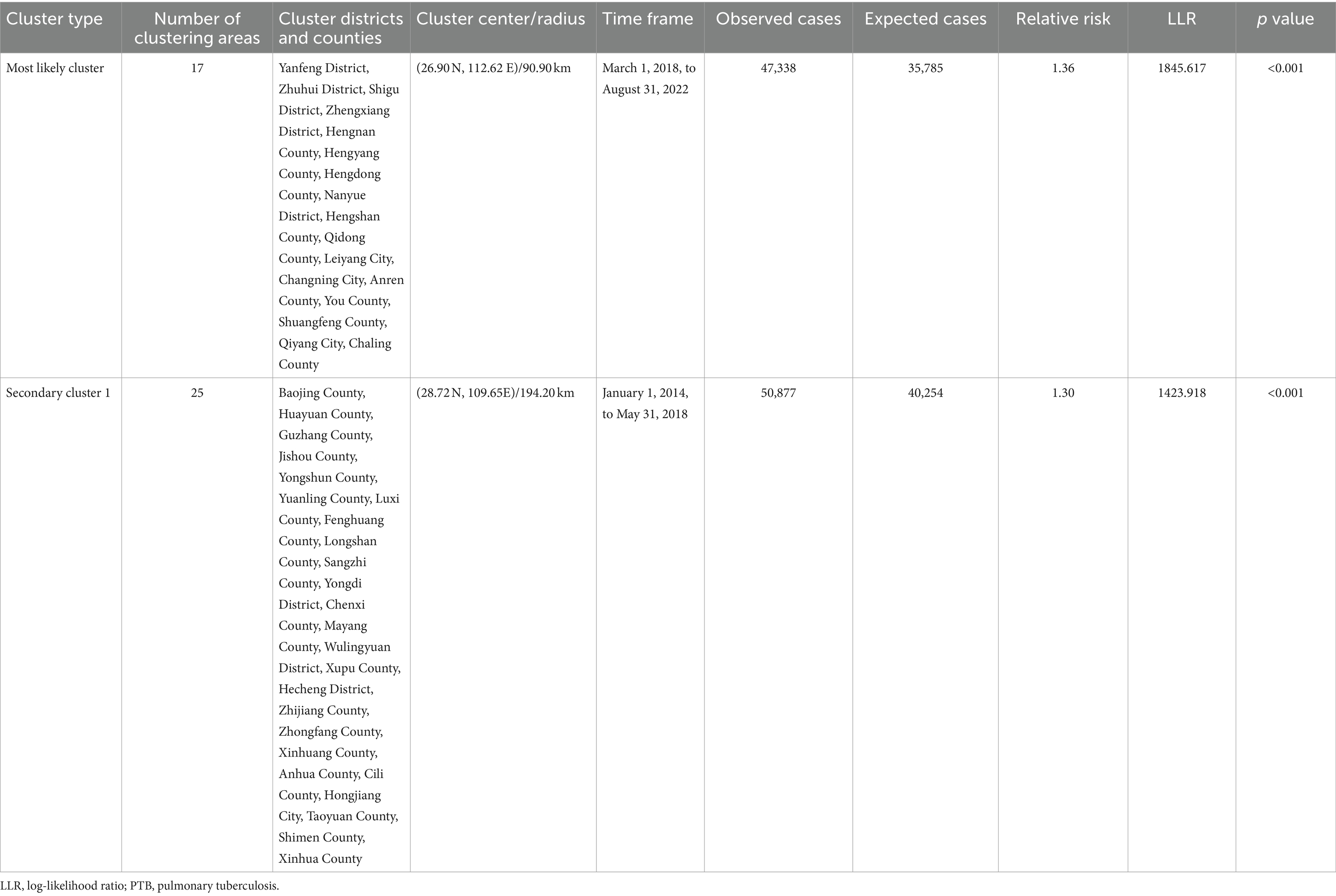
Table 3 . Space-time clustering of cases of PTB in the Hunan Province, China from January 2014 to December 2022.
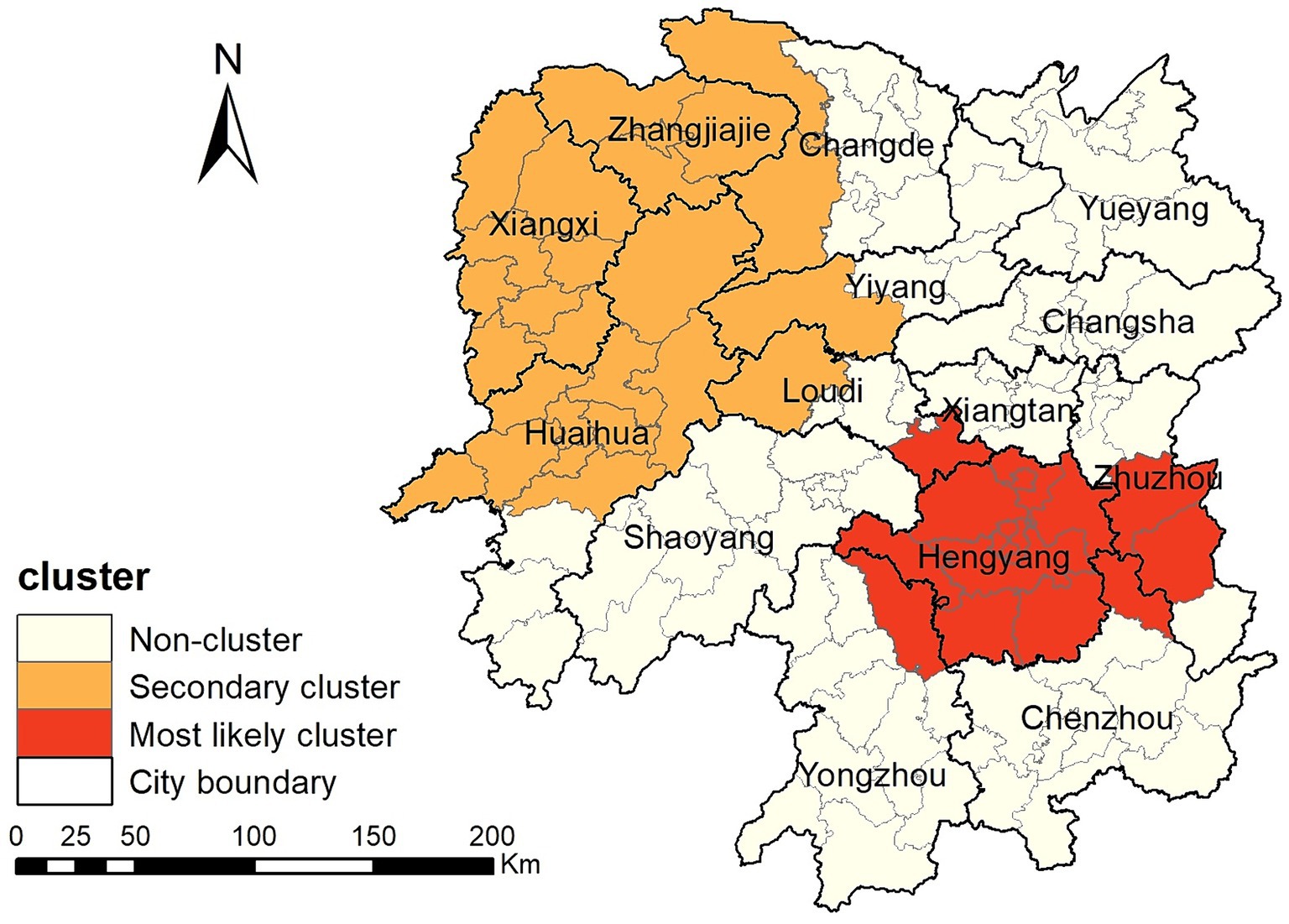
Figure 6 . Space-time clusters of cases of pulmonary tuberculosis at a county level in the Hunan Province from 2014 to 2022.
4 Discussion
This study found that the PTB notification rate decreased from 2014 to 2022 in the Hunan Province. PTB case notifications exhibited spatial clustering, with clustering occurring predominantly in the central-southern and northwestern regions of the province. The PTB notification rate in the Hunan Province decreased from 85.88/100,000 in 2014 to 66.40/100,000 in 2022, showing a slow downward trend with fluctuations. This declining trend mirrors observations made in various provinces and cities across China in a national study ( 26 ), including the Chongqing Municipality ( 13 ), Shandong Province ( 27 ), and Hubei Province ( 28 ). The decrease in the tuberculosis epidemic is mainly attributable to the efforts made by the national and local governments in tuberculosis control. The Hunan Province has implemented multiple measures to enhance its capacity for tuberculosis control across the province, establishing a novel tuberculosis control system. It actively conducts health education to raise awareness among the population about tuberculosis prevention and treatment. Molecular biology testing is widely promoted in prefectures and counties to achieve rapid diagnosis of PTB, leading to a gradual increase in the proportion of patients with microbiological confirmation and further improvement in the quality of tuberculosis diagnosis. Active screening is performed among key populations such as close contacts of patients with laboratory-confirmed PTB, older adults, and patients with diabetes patients to diagnose cases early, promptly initiate treatment, and reduce the spread of tuberculosis. In educational institutions, tuberculosis management has been strengthened by implementing PTB screening for students before entry into primary schools, middle schools, and high schools, as well as universities, controlling the entry of students with a high risk of developing tuberculosis. Additionally, preventive treatment for latent tuberculosis infection with a high risk of disease progression is promoted, reducing the occurrence of tuberculosis outbreaks in schools.
Our study found that the monthly PTB notifications showed significant seasonal variation, with peaks in January or March and troughs in December. The Hunan Province is located between 25° and 30° north latitude and has a subtropical monsoon climate. January is typically a winter month and is characterized by damp and rainy weather with little sunlight. Lack of exposure to ultraviolet light and poor ventilation are known risk factors for tuberculosis transmission ( 29 ). February coincides with the Chinese Lunar New Year holiday, when traditional customs discourage seeking medical care, leading to a higher PTB notification rate in March after the holiday period. This cyclical pattern is similar to research findings in the Chongqing Municipality ( 13 ), Hubei Province ( 28 ), Guangxi Province ( 30 ), and Hunan Province ( 15 ) but differs from the results observed in the Zhejiang Province ( 11 ), Taiwan ( 31 ) and Hong Kong ( 32 ). However, this pattern was disrupted from 2020 to 2022, with the peak in notifications delayed to June, April, and July in 2020, 2021, and 2022, respectively, although the trough still occurred in December each year. This may be attributed to the COVID-19 pandemic, which led to successive enforcement of control measures by the national and local governments based on the prevalence of COVID-19. People’s movements were affected, leading to delays in accessing healthcare services and diagnosis in individuals with PTB, thus disrupting the seasonal pattern of PTB notifications ( 33 , 34 ).
This study identified significant spatial clustering of PTB notifications in the Hunan Province, with hotspot areas primarily concentrated in the Xiangxi Autonomous Prefecture, Zhangjiajie City, and Hengyang City, similar to the findings of a previous study carried out in the Hunan Province in 2012 and 2013 ( 16 ). The hotspot areas in the Xiangxi Autonomous Prefecture gradually decreased over the years, disappearing by 2021 and 2022, whereas hotspot areas gradually formed in the Hengyang City from 2018 to 2022. Space-time scan analysis revealed that the most likely clustering area was around the Hengyang City, encompassing 17 adjacent counties and districts, with clustering occurring from March 1, 2018, to August 31, 2022. A secondary clustering area was identified around the Xiangxi Autonomous Prefecture and Zhangjiajie City, covering 25 adjacent counties and districts, with clustering occurring from January 1, 2014, to May 31, 2018. Local spatial autocorrelation analysis of hotspot areas was consistent with the clustering areas identified by space-time scan analysis, confirming the stability and reliability of the research results. The Xiangxi Autonomous Prefecture and Zhangjiajie City, located in the northwestern region of the province, are economically underdeveloped, with poor living conditions for residents and a lack of medical and health resources. Patients in these areas often come from impoverished families and may not receive timely diagnosis and treatment, causing the transmission of PTB and contributing to a higher incidence of the disease ( 35 , 36 ). In contrast, the Xiangxi Prefecture is an area in which ethnic minorities (Tujia and Miao) are concentrated. People belonging to ethnic minorities have an increased risk of delayed diagnosis of tuberculosis ( 37 ). In 2021, the PTB notification hotspot in the Zhangjiajie City decreased significantly, which may have been related to the COVID-19 epidemic in the Zhangjiajie City in July and August 2021 and the implementation of epidemic control measures. The COVID-19 pandemic resulted in reduced healthcare-seeking behavior among individuals with tuberculosis, affecting the diagnosis and treatment ( 34 , 38 ). This study also identified a cold-spot area that gradually expanded from the urban zones of Changsha, Zhuzhou, and Xiangtan in 2014 to encompass the entire city of Changsha, the urban zones of Zhuzhou and Xiangtan, and parts of Yueyang. This finding is generally similar to that of a previous study ( 15 ). These areas are the most economically developed region in the Hunan Province, with abundant medical resources and strong public awareness of disease prevention and treatment.
To our knowledge, this is the first space-time scan analysis of spatiotemporal clustering of PTB notifications at a county level in the Hunan Province. It identified regions with high PTB notification rates in the Hunan Province, providing valuable insights for formulating more effective control strategies.
This study has few limitations. First, the data were sourced from the National Disease Surveillance System, which may have resulted in an underestimation of the PTB epidemic in the Hunan Province due to some cases not being notified. Second, due to the adjustment of the national tuberculosis diagnostic criteria, cases of tuberculous pleurisy have been included in the pulmonary tuberculosis reporting system since May 1, 2018, which may introduce a slight bias in the research results. Third, this study used the space-time scan method to investigate PTB clustering. This method depends on circular spatial scan windows and space-time cylinders, which may not work well in irregular spaces, potentially leading to instability in determining clusters. Finally, this research examined only the spatiotemporal distribution patterns of tuberculosis without investigating individual factors and socioeconomic factors that may influence the incidence of tuberculosis. Some studies have found that personal factors such as smoking ( 39 ), alcohol consumption ( 40 , 41 ), HIV ( 42 ), and diabetes ( 43 ), as well as social factors such as poverty ( 36 ), population mobility ( 44 , 45 ), healthcare resources ( 46 ), and environmental pollution ( 47 , 48 ) may influence the distribution of tuberculosis. Future studies should aim to restrict the geographical unit of analysis to the township level and explore how these factors affect the incidence of PTB.
5 Conclusion
This study characterized the temporal trend and spatial distribution of tuberculosis in the Hunan Province. PTB diagnosis peaked in the spring. The PTB notification rate in the Hunan Province exhibited spatial clustering, concentrated mainly in the central-southern and northwestern regions of the province. This suggests that priority should be given to strengthening tuberculosis control efforts in these regions.
Data availability statement
The datasets presented in this article are not readily available because they are sourced from the Infectious Disease Information System and all data remain confidential. Requests to access the datasets should be directed to GH, [email protected] .
Ethics statement
The studies involving humans were approved by Medical Ethics Committee of Hunan Chest Hospital. The studies were conducted in accordance with the local legislation and institutional requirements. Written informed consent for participation was not required from the participants or the participants’ legal guardians/next of kin in accordance with the national legislation and institutional requirements.

Author contributions
GH: Data curation, Funding acquisition, Methodology, Software, Visualization, Writing – original draft. ZX: Data curation, Writing – review & editing. LB: Supervision, Writing – review & editing. JL: Conceptualization, Supervision, Writing – review & editing. SY: Software, Supervision, Writing – review & editing. HY: Methodology, Supervision, Writing – review & editing.
The author(s) declare that financial support was received for the research, authorship, and/or publication of this article. This study was supported by the Natural Science Foundation of Hunan Province (grant number: 2021JJ40281).
Acknowledgments
The authors thank the staff at all Centers for Disease Control and Prevention, tuberculosis-designated hospitals, and community healthcare centers in Hunan Province for implementing tuberculosis reporting and notification in their daily work.
Conflict of interest
The authors declare that the research was conducted in the absence of any commercial or financial relationships that could be construed as a potential conflict of interest.
Publisher’s note
All claims expressed in this article are solely those of the authors and do not necessarily represent those of their affiliated organizations, or those of the publisher, the editors and the reviewers. Any product that may be evaluated in this article, or claim that may be made by its manufacturer, is not guaranteed or endorsed by the publisher.
1. World Health Organization . Global Tuberculosis Report 2023 . Geneva: World Health Organization (2023).
Google Scholar
2. Xue, M, Zhong, J, Gao, M, Pan, R, Mo, Y, Hu, Y, et al. Analysis of spatial-temporal dynamic distribution and related factors of tuberculosis in China from 2008 to 2018. Sci Rep . (2023) 13:4974. doi: 10.1038/s41598-023-31430-0
Crossref Full Text | Google Scholar
3. Liu, MY, Li, QH, Zhang, YJ, Ma, Y, Liu, Y, Feng, W, et al. Spatial and temporal clustering analysis of tuberculosis in the mainland of China at the prefecture level, 2005–2015. Infect Dis Poverty . (2018) 7:106. doi: 10.1186/s40249-018-0490-8
4. Romanyukha, AA, Karkach, AS, Borisov, SE, Belilovsky, EM, Sannikova, TE, and Krivorotko, OI. Small-scale stable clusters of elevated tuberculosis incidence in Moscow, 2000–2015: Discovery and spatiotemporal analysis. Int J Infect Dis . (2020) 91:156–61. doi: 10.1016/j.ijid.2019.11.015
5. Lima, SVMA, Dos Santos, AD, Duque, AM, de Oliveira Goes, MA, da Silva Peixoto, MV, da Conceição, AD, et al. Spatial and temporal analysis of tuberculosis in an area of social inequality in Northeast Brazil. BMC Public Health . (2019) 19:873. doi: 10.1186/s12889-019-7224-0
6. Mesquita, CR, da Conceição, ML, de Oliveira, RAC, Conceição, EC, Garcez, JCD, Sousa, IFR, et al. Spatial analysis of tuberculosis patient flow in a neglected region of Northern Brazil. Trop Med Infect Dis . (2023):8. doi: 10.3390/tropicalmed8080397
7. Aceng, FL, Kabwama, SN, Ario, AR, Etwom, A, Turyahabwe, S, and Mugabe, FR. Spatial distribution and temporal trends of tuberculosis case notifications, Uganda: A ten-year retrospective analysis (2013–2022). BMC Infect Dis . (2024) 24:46. doi: 10.1186/s12879-023-08951-0
8. Huang, CC, Trevisi, L, Becerra, MC, Calderón, RI, Contreras, CC, Jimenez, J, et al. Spatial scale of tuberculosis transmission in Lima, Peru. Proc Natl Acad Sci USA . (2022) 119:e2207022119. doi: 10.1073/pnas.2207022119
9. Zhang, Y, Wang, XL, Feng, T, and Fang, CZ. Analysis of spatial-temporal distribution and influencing factors of pulmonary tuberculosis in China, during 2008–2015. Epidemiol Infect . (2018) 147:e25. doi: 10.1017/S0950268818002765
10. Li, L, Xi, Y, and Ren, F. Spatio-temporal distribution characteristics and trajectory similarity analysis of tuberculosis in Beijing, China. Int J Environ Res Public Health . (2016):13. doi: 10.3390/ijerph13030291
11. Ge, E, Zhang, X, Wang, X, and Wei, X. Spatial and temporal analysis of tuberculosis in Zhejiang Province, China, 2009–2012. Infect Dis Poverty . (2016) 5:11. doi: 10.1186/s40249-016-0104-2
12. Zhang, M, Chen, S, Luo, D, Chen, B, Zhang, Y, Wang, W, et al. Spatial-temporal analysis of pulmonary tuberculosis among students in the Zhejiang Province of China from 2007–2020. Front Public Health . (2023) 11:1114248. doi: 10.3389/fpubh.2023.1114248
13. Yu, Y, Wu, B, Wu, C, Wang, Q, Hu, D, and Chen, W. Spatial-temporal analysis of tuberculosis in Chongqing, China 2011–2018. BMC Infect Dis . (2020) 20:531. doi: 10.1186/s12879-020-05249-3
14. Rao, H, Shi, X, and Zhang, X. Using the Kulldorff’s scan statistical analysis to detect spatio-temporal clusters of tuberculosis in Qinghai Province, China, 2009–2016. BMC Infect Dis . (2017) 17:578. doi: 10.1186/s12879-017-2643-y
15. Alene, KA, Xu, Z, Bai, L, Yi, H, Tan, Y, Gray, DJ, et al. Spatiotemporal patterns of tuberculosis in Hunan Province, China. Int J Environ Res Public Health . (2021):18. doi: 10.3390/ijerph18136778
16. Zheng, J, Tang, Y, Zha, W, Liu, Y, Zhang, G, Hu, J, et al. Spatial distribution of tuberculosis in Hunan Province between 2012–2013: Analysis with geographic information system. Chin J Public Health . (2015) 31:1590–3. doi: 10.11847/zgggws2015-31-12-20
17. The People’s Government of Hunan Province, Introduction of Hunan . (2024). Available at: https://www.hunan.gov.cn/hnszf/jxxx/hngk/sqjs/sqjs.html (Accessed March 20, 2024).
18. National Health Commission of the People’s Republic of China . WS 288-2017 (2017). Beijing. Available at: http://www.nhc.gov.cn/cms-search/xxgk/getManuscriptXxgk.htm?id=0819ad84540b4d97a1644bbc6ec4306d (Accessed June 18, 2024).
19. National Health commission of the People’s Republic of China . Notice from the General Office of the National Health Commission on Adjusting the classification of infectious disease reports for pulmonary tuberculosis (2019). Beijing. Available at: http://www.nhc.gov.cn/jkj/s3589/201903/d779ae48db6446c28d1f5371ef09f5ab.shtml (Accessed March 1, 2024).
20. National Health commission of the People’s Republic of China . Notification of an adjustment to the classification of pulmonary tuberculosis reports (2017). Beijing. Available at: http://www.nhc.gov.cn/jkj/s3589/201707/e65698978c984013aa01b017f93dd75c.shtml (Accessed March 1, 2024).
21. Huo, XN, Li, H, Sun, DF, Zhou, LD, and Li, BG. Combining geostatistics with Moran’s I analysis for mapping soil heavy metals in Beijing, China. Int J Environ Res Public Health . (2012) 9:995–1017. doi: 10.3390/ijerph9030995
22. Anselin, L, Syabri, I, and Kho, Y. GeoDa: An introduction to spatial data analysis. Geogr Anal . (2006) 38:5–22. doi: 10.1111/j.0016-7363.2005.00671.x
23. Getis, A, and Ord, J. The analysis of spatial association by use of distance statistics. Geogr Analys . (1995) 27:93–115.
24. Kulldorff, M . A spatial scan statistic. Commun. Stat . (1997) 26:1481–96. doi: 10.1080/03610929708831995
25. Deng, T, Huang, Y, Yu, S, Gu, J, Huang, C, Xiao, G, et al. Spatial-temporal clusters and risk factors of hand, foot, and mouth disease at the district level in Guangdong Province, China. PLoS One . (2013) 8:e56943. doi: 10.1371/journal.pone.0056943
26. Dong, Z, Wang, QQ, Yu, SC, Huang, F, Liu, JJ, Yao, HY, et al. Age-period-cohort analysis of pulmonary tuberculosis reported incidence, China, 2006–2020. Infect Dis Poverty . (2022) 11:85. doi: 10.1186/s40249-022-01009-4
27. Duan, Y, Cheng, J, Liu, Y, Fang, Q, Sun, M, Cheng, C, et al. Epidemiological characteristics and spatial-temporal analysis of tuberculosis at the county-level in Shandong Province, China, 2016–2020. Trop Med Infect Dis . (2022) 7:346. doi: 10.3390/tropicalmed7110346
PubMed Abstract | Crossref Full Text | Google Scholar
28. Zhang, Y, Ye, J, Hou, S, Lu, X, Yang, C, Pi, Q, et al. Spatial-temporal analysis of pulmonary tuberculosis in Hubei Province, China, 2011–2021. PLoS One . (2023) 18:e0281479. doi: 10.1371/journal.pone.0281479
29. Thorpe, LE, Frieden, TR, Laserson, KF, Wells, C, and Khatri, GR. Seasonality of tuberculosis in India: Is it real and what does it tell us? Lancet . (2004) 364:1613–4. doi: 10.1016/S0140-6736(04)17316-9
30. Cui, Z, Lin, D, Chongsuvivatwong, V, Zhao, J, Lin, M, Ou, J, et al. Spatiotemporal patterns and ecological factors of tuberculosis notification: A spatial panel data analysis in Guangxi, China. PLoS ONE . (2019) 14:e0212051. doi: 10.1371/journal.pone.0212051
31. Liao, CM, Hsieh, NH, Huang, TL, Cheng, YH, Lin, YJ, Chio, CP, et al. Assessing trends and predictors of tuberculosis in Taiwan. BMC Public Health . (2012) 12:29. doi: 10.1186/1471-2458-12-29
32. Leung, CC, Yew, WW, Chan, TYK, Tam, CM, Chan, CY, Chan, CK, et al. Seasonal pattern of tuberculosis in Hong Kong. Int J Epidemiol . (2005) 34:924–30. doi: 10.1093/ije/dyi080
33. Zhang, Y, Zhang, L, Gao, W, Li, M, Luo, Q, Xiang, Y, et al. The impact of COVID-19 pandemic on reported tuberculosis incidence and mortality in China: An interrupted time series analysis. J Glob Health . (2023) 13:06043. doi: 10.7189/jogh.13.06043
34. Xu, J, Wang, Y, Liu, F, and Yang, H. Changes of tuberculosis infection in mainland China before and after the COVID-19 pandemic. J Infect . (2023) 86:154–225. doi: 10.1016/j.jinf.2022.12.008
35. Mou, J, Griffiths, SM, Fong, H, and Dawes, MG. Health of China’s rural-urban migrants and their families: A review of literature from 2000 to 2012. Br Med Bull . (2013) 106:19–43. doi: 10.1093/bmb/ldt016
36. Huang, L, Li, XX, Abe, EM, Xu, L, Ruan, Y, Cao, CL, et al. Spatial-temporal analysis of pulmonary tuberculosis in the northeast of the Yunnan Province, People’s Republic of China. Infect Dis Poverty . (2017) 6:53. doi: 10.1186/s40249-017-0268-4
37. Gilmour, B, Xu, Z, Bai, L, Alene, KA, and Clements, ACA. The impact of ethnic minority status on tuberculosis diagnosis and treatment delays in Hunan Province China. BMC Infect Dis . (2022) 22:90. doi: 10.1186/s12879-022-07072-4
38. Zhang, G, Yu, Y, Zhang, W, Shang, J, Chen, S, Pang, X, et al. Influence of COVID-19 for delaying the diagnosis and treatment of pulmonary tuberculosis-Tianjin, China. Front Public Health . (2022) 10:937844. doi: 10.3389/fpubh.2022.937844
39. Feng, Y, Xu, Y, Yang, Y, Yi, G, Su, H, Chen, H, et al. Effects of smoking on the severity and transmission of pulmonary tuberculosis: A hospital-based case control study. Front Public Health . (2023) 11:1017967. doi: 10.3389/fpubh.2023.1017967
40. Weiangkham, D, Umnuaypornlert, A, Saokaew, S, Prommongkol, S, and Ponmark, J. Effect of alcohol consumption on relapse outcomes among tuberculosis patients: A systematic review and meta-analysis. Front Public Health . (2022) 10:962809. doi: 10.3389/fpubh.2022.962809
41. Wigger, GW, Bouton, TC, Jacobson, KR, Auld, SC, Yeligar, SM, and Staitieh, BS. The impact of alcohol use disorder on tuberculosis: A review of the epidemiology and potential immunologic mechanisms. Front Immunol . (2022) 13:864817. doi: 10.3389/fimmu.2022.864817
42. Zou, S, Tan, Y, Xiang, Y, Liu, Y, Zhu, Q, Wu, S, et al. The role of CD4+CD8+ T cells in HIV infection with tuberculosis. Front Public Health . (2022) 10:895179. doi: 10.3389/fpubh.2022.895179
43. Ssekamatte, P, Sande, OJ, van Crevel, R, and Biraro, IA. Immunologic, metabolic and genetic impact of diabetes on tuberculosis susceptibility. Front Immunol . (2023) 14:1122255. doi: 10.3389/fimmu.2023.1122255
44. Zhang, H, Sun, R, Wu, Z, Liu, Y, Chen, M, Huang, J, et al. Spatial pattern of isoniazid-resistant tuberculosis and its associated factors among a population with migrants in China: A retrospective population-based study. Front Public Health . (2024) 12:1372146. doi: 10.3389/fpubh.2024.1372146
45. Liu, K, Chen, S, Zhang, Y, Li, T, Xie, B, Wang, W, et al. Tuberculosis burden caused by migrant population in Eastern China: Evidence from notification records in Zhejiang Province during 2013–2017. BMC Infect Dis . (2022) 22:109. doi: 10.1186/s12879-022-07071-5
46. Pradipta, IS, Idrus, LR, Probandari, A, Puspitasari, IM, Santoso, P, Alffenaar, JC, et al. Barriers to optimal tuberculosis treatment services at community health centers: A qualitative study from a high prevalent tuberculosis country. Front Pharmacol . (2022) 13:857783. doi: 10.3389/fphar.2022.857783
47. Nardell, EA . Indoor environmental control of tuberculosis and other airborne infections. Indoor Air . (2016) 26:79–87. doi: 10.1111/ina.12232
48. Li, JX, Luan, Q, Li, B, Dharmage, SC, Heinrich, J, Bloom, MS, et al. Outdoor environmental exposome and the burden of tuberculosis: Findings from nearly two million adults in northwestern China. J Hazard Mater . (2023) 459:132222. doi: 10.1016/j.jhazmat.2023.132222
Keywords: tuberculosis, spatiotemporal analysis, spatial autocorrelation analysis, clustering, China
Citation: Huang G, Xu Z, Bai L, Liu J, Yu S and Yao H (2024) Spatiotemporal analysis of tuberculosis in the Hunan Province, China, 2014–2022. Front. Public Health . 12:1426503. doi: 10.3389/fpubh.2024.1426503
Received: 01 May 2024; Accepted: 30 July 2024; Published: 08 August 2024.
Reviewed by:
Copyright © 2024 Huang, Xu, Bai, Liu, Yu and Yao. This is an open-access article distributed under the terms of the Creative Commons Attribution License (CC BY) . The use, distribution or reproduction in other forums is permitted, provided the original author(s) and the copyright owner(s) are credited and that the original publication in this journal is cited, in accordance with accepted academic practice. No use, distribution or reproduction is permitted which does not comply with these terms.
*Correspondence: Hongyan Yao, [email protected]
Disclaimer: All claims expressed in this article are solely those of the authors and do not necessarily represent those of their affiliated organizations, or those of the publisher, the editors and the reviewers. Any product that may be evaluated in this article or claim that may be made by its manufacturer is not guaranteed or endorsed by the publisher.
Surgical repair of Rasmussen aneurysm of left pulmonary artery—case report
- Case report
- Published: 10 August 2024
Cite this article
- Shyam Sundar Rengan 1 ,
- Preksha Rani ORCID: orcid.org/0009-0005-3094-1033 1 ,
- Rohit Kumar Rathi 1 ,
- Haritha Therese Joseph 1 ,
- Abhishek Mohan 1 &
- Sabyasachi Bal 1
Rasmussen aneurysm refers to the aneurysmal dilatation of a pulmonary arterial branch adjacent to a cavitatory lesion caused by tuberculosis (TB). It is a rare occurrence and is predominantly treated using endovascular techniques. Here, we present a case of Rasmussen aneurysm involving the left pulmonary artery, which was surgically managed on an emergent basis, with a successful outcome. Upon conducting an English literature search, using Google Scholar and PubMed database with MeSH words “rasmussen aneurysm,” “main pulmonary artery AND aneurysm AND Rasmussen,” “massive hemoptysis tuberculosis,” pulmonary artery aneurysm AND tuberculosis, we found no other case reports of Rasmussen aneurysm originating from such a central vascular location.
This is a preview of subscription content, log in via an institution to check access.
Access this article
Subscribe and save.
- Get 10 units per month
- Download Article/Chapter or eBook
- 1 Unit = 1 Article or 1 Chapter
- Cancel anytime
Price includes VAT (Russian Federation)
Instant access to the full article PDF.
Rent this article via DeepDyve
Institutional subscriptions
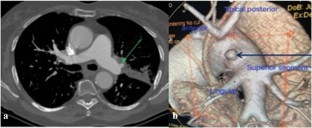
Data availability
Not applicable.
Code availability
Abbreviations.
- Tuberculosis
Computed tomography
Three dimensional
Marak JR, Kumar T, Gara H, Dwivedi S. Rasmussen aneurysm: case series of a rare complication of pulmonary tuberculosis. Respir Med Case Rep. 2023;45:101897.
PubMed PubMed Central Google Scholar
Mahmoud N, Moussa C, Attia M, Rouis H, Khattab A, Khouaja I, et al. Rasmussen aneurysm: a forgotten complication of tuberculosis in the COVID-19 era. Respir Med Case Rep. 2022;39: 101714.
World Health Organization. Global tuberculosis report 2021: supplementary material. World Health Organization; 2022 Mar 31. https://iris.who.int/handle/10665/360605 . Accessed on 13 Jun 2024.
Dhamnetiya D, Patel P, Jha RP, Shri N, Singh M, Bhattacharyya K. Trends in incidence and mortality of tuberculosis in India over past three decades: a joinpoint and age–period–cohort analysis. BMC Pulm Med. 2021;21:1–4.
Article Google Scholar
Seedat UF, Seedat F. Post-primary pulmonary TB haemoptysis–when there is more than meets the eye. Respir Med Case Rep. 2018;25:96–9.
Kidd P. Unusual cases of pulmonary artery aneurysm. Trans Pathol Soc Lond. 1884;35:98–100.
Google Scholar
Picard C, Parrot A, Boussaud V, Lavolé A, Saidi F, Mayaud C, et al. Massive hemoptysis due to Rasmussen aneurysm: detection with helicoidal CT angiography and successful steel coil embolization. Intensive Care Med. 2003;29:1837–9.
Article PubMed Google Scholar
Park HS, Chamarthy MR, Lamus D, Saboo SS, Sutphin PD, Kalva SP. Pulmonary artery aneurysms: diagnosis & endovascular therapy. Cardiovasc Diagn Ther. 2018;8:350.
Article PubMed PubMed Central Google Scholar
Yazgan S, Ucvet A, Ceylan K, Heskiloglu C, Yoldas B, Sevinc S. Emergency pulmonary resection in massive hemoptysis: analysis of 39 patients. Kardiochir Torakochirurgia Pol. 2021;18:203–9.
PubMed Google Scholar
Download references
No source of funding.
Author information
Authors and affiliations.
Institute of Chest Surgery, Chest Surgical Oncology, Minimally Invasive & Robotic Chest Surgery, Sir Ganga Ram Hospital, New Delhi, India
Shyam Sundar Rengan, Preksha Rani, Rohit Kumar Rathi, Haritha Therese Joseph, Abhishek Mohan & Sabyasachi Bal
You can also search for this author in PubMed Google Scholar
Contributions
Shyam Rengan, Preksha Rani, and Rohit Kumar Rathi took part in writing the article, researching the bibliography, and taking care of the patient. Haritha Joseph and Abhishek Kumar took part in taking photos and taking care of the patient. Sabyasachi Bal participated in the design and final approval and submission of the manuscript.
Corresponding author
Correspondence to Preksha Rani .
Ethics declarations
Conflict of interest.
None of the authors declares any conflict of interest.
Ethics approval
The approval of the Institutional Review Board is not required for a case report.
Consent to participate
Written consent for the case study and publication was obtained from the patient.
Statement of Human and animal rights
Additional information, publisher's note.
Springer Nature remains neutral with regard to jurisdictional claims in published maps and institutional affiliations.
Rights and permissions
Springer Nature or its licensor (e.g. a society or other partner) holds exclusive rights to this article under a publishing agreement with the author(s) or other rightsholder(s); author self-archiving of the accepted manuscript version of this article is solely governed by the terms of such publishing agreement and applicable law.
Reprints and permissions
About this article
Rengan, S.S., Rani, P., Rathi, R.K. et al. Surgical repair of Rasmussen aneurysm of left pulmonary artery—case report. Indian J Thorac Cardiovasc Surg (2024). https://doi.org/10.1007/s12055-024-01782-x
Download citation
Received : 26 March 2024
Revised : 13 June 2024
Accepted : 14 June 2024
Published : 10 August 2024
DOI : https://doi.org/10.1007/s12055-024-01782-x
Share this article
Anyone you share the following link with will be able to read this content:
Sorry, a shareable link is not currently available for this article.
Provided by the Springer Nature SharedIt content-sharing initiative
- Rasmussen aneurysm
- Pulmonary artery aneurysm
- Find a journal
- Publish with us
- Track your research

An official website of the United States government
The .gov means it’s official. Federal government websites often end in .gov or .mil. Before sharing sensitive information, make sure you’re on a federal government site.
The site is secure. The https:// ensures that you are connecting to the official website and that any information you provide is encrypted and transmitted securely.
- Publications
- Account settings
Preview improvements coming to the PMC website in October 2024. Learn More or Try it out now .
- Advanced Search
- Journal List
- Cold Spring Harb Perspect Med
- v.6(1); 2016 Jan
Clinical Aspects of Adult Tuberculosis
Robert loddenkemper.
1 Charité Universitätsmedizin Berlin, Department of Pneumology, HELIOS-Klinikum Emil von Behring, 14165 Lungenklinik Heckeshorn, Berlin, Germany
Marc Lipman
2 Respiratory & HIV Medicine, Royal Free London NHS Foundation Trust, University College London, London NW3 2QG, United Kingdom
Alimuddin Zumla
3 Division of Infection and Immunity, University College London, Consultant Infectious Diseases Physician, University College London Hospitals NHS Foundation Trust, London NW3 2PF, United Kingdom
Tuberculosis (TB) in adults can present in a large number of ways. The lung is the predominant site of TB. Primary pulmonary TB should be distinguished from postprimary pulmonary TB, which is the most frequent TB manifestation in adults (70%–80% cases). Cough is common, although the chest radiograph often raises suspicion of disease. Sputum sampling is a key step in the diagnosis of TB, and invasive procedures such as bronchoscopy may be necessary to achieve adequate samples for diagnosis. Extrapulmonary involvement, which may present many years after exposure, occurs in a variable proportion of cases (20%–45%). This reflects the country of origin of patients and also the frequency of associated human immunodeficiency virus (HIV) coinfection. In the latter case, the presentation of TB is often nonspecific, and care needs to be taken to not miss the diagnosis. Anti-TB therapy should be given in line with proven (or assumed) drug resistance. In extrapulmonary TB, adjunctive therapeutic measures may be indicated; although in all cases, support is often required to ensure that people are able to complete treatment with minimal adverse events and maximal adherence to the prescribed regimen, and so reduce risk of future disease for themselves and others.
Postprimary tuberculosis (TB) of the lungs is the prevailing TB manifestation in adults. It may spread from the lungs via the lymphatics or bloodstream to other body sites, causing various extrapulmonary TB manifestations.
In parts of the world where tuberculosis (TB) is endemic, symptomatic individuals and their health-care providers are likely to recognize and consider TB early within the diagnostic algorithm ( Aït-Khaled et al. 2010 ). However, in countries with a declining or low prevalence, the time to diagnosis and starting treatment can be prolonged. This may be attributable to both patient and health system factors ( Millen et al. 2008 , Migliori et al. 2012 ) and can be accentuated by the stigma associated with a potential diagnosis of TB (as well as HIV coinfection) ( Courtwright and Turner 2010 ). Hence, education for individuals, communities, and social care providers about TB symptoms, and where and how to get them investigated, is an important part of clinical management ( Chowdhury et al. 2013 ; TB CARE 2014 ).
Considering TB as the cause of a person’s symptoms (or new signs in a child with a significant exposure), making a rapid and accurate diagnosis and initiating effective treatment promptly are central to good patient care and public health TB management. Not only does this alleviate symptoms and reduce mortality for the individual, but it also decreases onward transmission to others and hence impacts on the overall burden of TB.
TB in adults can present in a variety of ways. The lung is the predominant site of TB as infection with Mycobacterium tuberculosis ( Mtb ) or other members of the TB complex arises almost exclusively from inhaling droplets containing the bacilli. This may result in symptomatic, primary pulmonary TB disease (usually in children) and in adults, after a variable amount of time in a clinically asymptomatic state of latent TB infection (LTBI), generally as postprimary pulmonary TB. Mtb may spread directly from the lungs, via the lymphatics, or the bloodstream, to other body sites causing the various extrapulmonary TB manifestations described in this review.
The location of disease should be documented in all patients. Given that there may be multiple sites involved, it is recommended that at least two, a major and a minor site, when applicable, be recorded. Here, the definitions used are those proposed by a Working Group of the World Health Organization (WHO) and the European Region of the International Union Against Tuberculosis and Lung Disease (IUATLD) for uniform reporting of TB cases ( Rieder et al. 1996 ).
It is important to note that TB can be diagnosed via a number of different clinical pathways and settings. In a low incidence country such as Germany, among more than 25,000 TB cases studied between 1996 and 2000, almost 80% were diagnosed through passive case finding (∼62% had symptoms suggesting TB, 16% were diagnosed during investigations for other medical causes, 1% at autopsy) and 19% by active case finding in high-risk groups, particularly in close contacts of infectious patients ( Forssbohm 2004 ). This further reinforces the importance of thinking of TB as a possible cause of a patient’s symptoms or signs in clinical practice ( Craig et al. 2009 ).
CLINICAL PRESENTATION AND DIAGNOSIS OF TB
Pulmonary tb.
Pulmonary TB is defined as tuberculosis of the lung parenchyma and the tracheobronchial tree only. Primary pulmonary TB should be distinguished from postprimary pulmonary TB, which is the most frequent TB manifestation in adults. The classic clinical features of pulmonary TB include chronic cough, sputum production, appetite loss, weight loss, fever, night sweats, and hemoptysis ( Lawn and Zumla 2011 ). Someone presenting with any of these symptoms should be suspected of having TB. If they are or were known to be in contact with infectious TB, they are even more likely to be suffering from TB ( Ait-Khaled et al. 2010 .)
Primary Pulmonary TB
In countries with a high TB prevalence, primary pulmonary disease occurs usually in childhood, but where TB is less endemic, it occurs fairly often also in adults. It is characterized by local granulomatous inflammation, usually in the periphery of the lung (Ghon focus), and may be accompanied by ipsilateral lymph node involvement, termed the Ghon complex. The infection is usually asymptomatic but can present as an acute lower respiratory tract infection. The most important clue to the diagnosis is a history of close contact with an infectious TB case. The diagnosis is suspected when a tuberculin skin test or a blood interferon-γ release assay (IGRA) converts to positive, usually 3–8 wk after infection. The chest radiograph may show the Ghon focus/complex ( Fig. 1 ).

Ghon complex. (Figure reprinted from Fuehner et al. 2007 , with permission, from Springer, © 2007.)
Rare primary sites of TB are the alimentary tract caused by swallowing Mtb , usually Mycobacterium bovis, present in nonpasteurized milk products ( de la Rua-Domenech 2006 ), or after direct cutaneous infection (usually occurring in laboratory personnel) ( Menzies et al. 2003 ). Intravesical Bacillus Calmette–Guérin (BCG) vaccination used to treat localized bladder tumors may occasionally disseminate and present as primary BCG disease ( Fig. 2 ) ( Lamm 1992 ).

Disseminated BCG disease. Computed tomography (CT) of the chest showing bilateral patchy ground glass shadowing and consolidation in a patient who developed fevers, cough, and positive blood cultures for BCG following treatment with intravesical BCG for bladder carcinoma. (Figure provided by Marc Lipman.)
Local complications of primary pulmonary TB may result from lymph node enlargement leading to bronchial obstruction. Tuberculous pleurisy can arise early in the course of primary pulmonary TB, either by direct spread from the pulmonary lesion or through hematogenous dissemination.
Rare, serious, early systemic complications caused by blood-borne spread of Mtb are miliary TB and meningitis. Many years later, TB of the skeletal or urogenital system and other organs may result ( Wallgren 1948 ).
Postprimary pulmonary TB may follow primary TB. In the generally immunocompetent, there is a lifetime chance of reactivation of the dormant primary complex of 5%–10% ( Horsburgh Jr 2004 ). These estimates were developed before the availability of molecular techniques that can distinguish reactivation from reinfection with another strain of Mtb , and it may be that the overall risk is rather less in most people with latent TB infection not exposed again to Mtb . The first 2 yr following primary infection are the period of maximal risk of progression. This can be reduced significantly by treating LTBI, which is indicated particularly in high-risk groups ( Table 1 ) ( Diel et al. 2013 ). It is not known why only ∼10% of individuals infected with Mtb develop active disease. Apart from diverse risk factors such as diabetes, smoking, and chronic renal failure ( Hu et al. 2014 ), several genes have been found to be associated with increased susceptibility to, or resistance against, Mtb ( Möller et al. 2010 ).
Persons at high risk of progressing from latent to active TB
Recent contact with a TB case |
Persons with fibrotic changes on chest radiograph consistent with old TB |
HIV-infected persons |
Organ transplant recipients |
Persons immunosuppressed for other reasons (e.g., taking the equivalent of 15 mg/d of prednisone for >1 mo or taking tumor necrosis factor-α inhibitors) |
Recent immigrants (<5 yr) from high prevalence countries |
Persons with the following clinical conditions: |
Diabetes mellitus |
Chronic renal failure |
Some hematologic disorders (e.g., leukemia and lymphomas) |
Other specific malignancies (e.g., carcinoma of the head or neck and lung) |
Gastrectomy and jejunoileal bypass |
Silicosis |
Injection drug users |
Tobacco smokers |
Residents and employees of high-risk congregate living facilities (e.g., correctional facilities, nursing homes, homeless shelters, hospitals, and other health-care facilities) |
Mycobacteriology laboratory personnel |
Data modified from Diel et al. 2013.
Erythema nodosum and other skin conditions such as granulomatous panniculitis plus forms of uveitis and polyarthritis (Poncet's disease) are considered to reflect the host immune response to mycobacterial antigen. They can be seen both during primary infection and at some time distant from presumed infection ( Kroot et al. 2007 ). The features are often nonspecific, and the key clinical issue here is to consider TB as a possible underlying cause of the presentation. This is generally based on determining whether there is a history of exposure or residence in a TB-endemic area. The increasing numbers of individuals who are immunocompromised from infections such as HIV or through medical treatments (and are at much greater risk of developing active TB) make this method of categorization less helpful than previously.
Postprimary Pulmonary TB
Postprimary TB of the lungs is the prevailing TB manifestation in adults (in 60%–80%) ( Public Health England 2014 ). It can occur many years after exposure to an individual with infectious TB and may be provoked by temporary or permanent immunological impairment. Males are more often affected than females ( Borgdorff et al. 2000 ).
The most frequent symptoms of active disease are fever, anorexia or reduced appetite, weight loss, night sweats, anemia, and persistent cough (i.e., lasting >14 d) ( TB CARE 2014 ) usually productive of purulent and/or blood-stained sputum. Occasionally, patients complain of localized thoracic pain attributable to accompanying pleural inflammation. In extensive and long-lasting pulmonary disease, patients may report breathlessness. Hemoptysis is usually the result of cavitating lung disease causing erosion of pulmonary blood vessels ( Fig. 3 ).

Cavitating tuberculosis presenting with hemoptysis (note arrows indicating cavities). (Figure reprinted from Fuehner et al. 2007 , with permission, from Springer, © 2007.)
Hoarseness can occur if there is laryngeal involvement. Patients with laryngeal and tracheobronchial ( Fig. 4 ) TB may have Mtb in sputum despite a normal chest X-ray, although in general, the radiograph will suggest the possible diagnosis ( Bhat et al. 2009 ).

TB in the mucosa of the left main bronchus. Histology and culture from biopsy were positive for Mtb . (Figure provided by Robert Loddenkemper.)
Tuberculoma of the lung should be considered as part of the differential diagnosis in mass lesions presenting in individuals with a history of exposure to Mtb ( Fig. 5 ).

Lung tuberculoma. Initially thought to be a primary lung cancer, bronchoscopic biopsy showed acid-fast bacilli on smear. Culture grew Mtb . (Figure provided by Marc Lipman.)
The physical signs of TB are not specific. Auscultation often does not show any pathological breath sounds or may reveal crackles, wheezing, or bronchial breathing (which is termed amphoric when related to the movement of air within a cavity). The radiographic features vary from very discrete infiltrates to extensive and bilateral changes with multiple cavities characteristic of TB. Usually, the changes are more pronounced in the upper lobes. The differential diagnosis of cavitation includes other infections as well as malignant and inflammatory lung diseases ( Table 2 ).
Differential diagnosis of pulmonary cavitation
Cause | Examples |
---|---|
Infection | Tuberculosis |
Acute bacterial, e.g., , | |
Subacute/chronic bacterial (e.g., sp, melidoidosis, actinomycosis) | |
Fungal (e.g., cryptococcosis, coccidiodomycosis) | |
Infected pneumatocele | |
Malignancy | Primary lung cancer (e.g., non–small cell squamous) |
Pulmonary metastases | |
Inflammatory | Sarcoidosis |
Wegener's granulomatous angiitis | |
Rheumatoid arthritis | |
Vascular | Pulmonary infarct |
Congenital | Sequestered lung; bronchogenic cyst |
There are no specific blood tests that aid the diagnosis of TB. Patients will have a profile that reflects their general state of health and nutrition. They may or may not be anemic and have moderately elevated inflammatory markers such as C-reactive protein. However, all of these measures are highly variable ( Breen et al. 2008a ). The following blood tests are recommended generally as baseline assessments rather than for any specific value in TB diagnosis. These include kidney and liver function, blood sugar, hemoglobin, white cell and differential counts, platelet count, and C-reactive protein (or ESR). It is recommended that routine HIV and hepatitis B and C testing be performed and vitamin D blood levels measured, as patients are invariably deficient ( Blasi and Matteelli 2013 ).
The presentation of HIV-associated pulmonary TB can mimic that seen in the HIV uninfected. This is generally found in subjects with relatively well-preserved immunity (manifest as blood CD4 counts >350 cells/μL) ( Cain et al. 2010 ). However, the associated immune dysregulation in most individuals with active TB and HIV coinfection means that although symptoms may be similar, patients can also present with very nonspecific although severe systemic features (e.g., weight loss, night sweats, and fever) and minimal specific respiratory complaints ( Breen et al. 2008a ).
The radiology of HIV TB is discussed in more detail elsewhere. However, an important point to note is that parenchymal cavitation is less likely in individuals with low blood CD4 counts. Extrapulmonary manifestations (including mediastinal nodal disease and pleural effusions) are also more common in HIV coinfection ( Lawn and Zumla 2011 ).
The lack of cavities means that sputum samples in HIV-associated pulmonary TB are often smear-negative. This is despite individuals having in fact a very high mycobacterial burden ( Elliott et al. 1993 ; Crump et al. 2012 ; Huang et al. 2014 ). Indeed, sputum cultures are generally positive as frequently in HIV-infected as -uninfected subjects. The importance of obtaining samples from other body fluids and locations cannot be overemphasized. This should include mycobacterial blood and urine cultures. Given that HIV-associated TB is often multisite, general radiological evaluation (including computed tomography [CT] chest, abdomen, and pelvis or equivalent investigations, e.g., abdominal ultrasound) may identify other areas of pathology that are amenable to diagnostic aspiration or biopsy. The high mycobacterial burden associated with HIV means that often urine antigen tests such as the cell wall component lipoarabinnoman (available in commercially produced kits) may be positive in patients with very low blood CD4 counts (<100 cells/μL). These offer, therefore, an alternative approach to TB diagnosis in advanced HIV infection ( Lawn et al. 2013 ).
In many clinical settings, HIV is diagnosed at the same time as TB ( Rice et al. 2013 ). This mainly follows the pattern of presentation with clinical TB, which then results in an HIV test. However, the use of antiretroviral therapy in an individual at risk of TB can lead to an unmasking paradoxical reaction (which is part of the immune reconstitution inflammatory syndrome, IRIS) ( Breen et al. 2005 ). These are typically highly inflammatory presentations, in which individuals develop intense systemic symptoms and new onset signs and radiological changes within a matter of days.
Although the underlying mechanism has not been fully elucidated, it appears to arise as a consequence of a host immune response directed against a (generally foreign) persistent antigen. Mycobacteria fulfill this role well, although so do other chronic intracellular organisms such as fungi. Hence, a presentation of apparent unmasking TB must be investigated comprehensively. Samples should be sent for cytology, histology, and bacterial, fungal, and mycobacterial cultures to avoid diagnostic confusion and inappropriate treatment.
Sputum sampling is a key step in the diagnosis of TB. Attempts should be made to obtain at least two sputum samples from individuals with suspected TB (including extrapulmonary TB, which is often associated with positive sputum cultures despite few reported symptoms) ( Parimon et al. 2008 ). Good-quality sputum samples should be obtained. It is standard to ask for three samples, although evidence suggests that two may strike a better balance in terms of value ( Mase et al. 2007 ). These are generally requested to be provided first thing in the morning (i.e., at a time when individuals are most productive). However, in an ambulatory setting, this can result in delay, and often there is utility in obtaining samples in one day (e.g., in the morning and afternoon).
Should an individual be unable to produce sputum spontaneously, many centers will perform induced sputum using hypertonic saline (generally between 3% and 7% concentration). This technique is quick (in total taking <1 h), relatively inexpensive, and not as invasive as a bronchoscopy. It also has a good yield ( Brown et al. 2007 ). However, this is operator-dependent, and the procedure requires experienced technicians to ensure that optimal samples are obtained.
Sputum induction creates a considerable aerosol of potentially infectious material ( Dooley et al. 1990 ). It should therefore be performed in a setting where infection control is of a high standard, or there is minimal risk of transmission to others, e.g., induction performed in an open air setting, away from others.
Should sputum induction be unavailable or unsuccessful, flexible bronchoscopy with bronchoalveolar lavage is indicated. This procedure has the advantage of being able to sample specific sites (and hence in theory improve the recovery from, e.g., localized disease or a cavity) ( Schoch et al. 2007 ). A good-quality sample, which is promptly set up for mycobacterial culture, has a high diagnostic yield ( Breen et al. 2008b ). This is important because the procedure is associated with significant up-front capital cost, and potential risk to both patients and health-care workers.
Bronchoscopic procedures also enable aspiration and biopsies to be performed. This includes both lung tissue (bronchial biopsies) and needle aspiration under either direct vision or using ultrasound (EBUS) ( Navani et al. 2011 ).
Pulmonary samples can be assessed using acid-fast bacilli (AFB) smear, nucleic acid amplification techniques such as GeneXpert, mycobacterial culture, or cytological analysis. AFB sputum smear is the most long standing, although it is fairly insensitive (requiring at least 5 × 10 3 bacilli per mL for detection). Molecular diagnostics are equally rapid, more sensitive, and also currently more expensive. They can also detect genetic resistance to antituberculosis drugs ( Boehme et al. 2011 ), and with modification they might distinguish between live and dead bacilli ( Honeyborne et al. 2011 ).
Culture techniques are still regarded as the gold standard. Liquid fluorometric systems are faster than solid media and more sensitive ( Dhillon et al. 2014 ). However, they are also expensive and require skilled personnel to use them. At present, they are not routinely available worldwide for all patient samples that are obtained for suspected TB.
Extrapulmonary Tuberculosis
Extrapulmonary tuberculosis (EPTB) occurs in 10%–42% of adult TB patients depending on race or ethnic background, age, presence or absence of underlying disease, genotype of the Mtb strain, and immune status ( Caws et al. 2008 ) ( Table 3 ). EPTB is defined as TB affecting any site other than the lung. Sometimes it is accompanied by pulmonary disease ( Public Health England 2014 ). It can affect any organ in the body and has numerous clinical manifestations, and thus requires a high index of clinical suspicion ( Zumla et al. 2013 ). EPTB can occur many years after exposure. In addition to systemic features, patients with EPTB may complain of symptoms related to the involved site/organ. In contrast to pulmonary TB, females are more often affected (one exception to this being pleurisy) ( Forssbohm et al. 2008 ) ( Fig. 6 ). The differences in the proportion of EPTB by area of origin are remarkable. Patients from Africa and Asia are generally far more likely to present with EPTB than those from Germany ( Forssbohm et al. 2008 ); genetic factors may explain some of these differences ( Fernando et al. 2007 ). HIV and other conditions that markedly impair host immunity have led to an increase in extrapulmonary disease. This reflects an inability to contain Mtb , and hence dissemination, with positive cultures from a number of body sites, often occurs. The diagnosis can require biopsy and/or surgical removal.

Age-specific female-to-male odds ratio (OR) of having extrapulmonary TB compared with pulmonary TB by site of extrapulmonary TB, Germany 1996–2000. Solid circle, lymphatic, peripheral; open circle, lymphatic intrathoracic; solid square, pleural; open square, genitourinary; solid triangle, osteoarticular; inverted solid triangle, meningeal; inverted open triangle, peritoneal. (Figure reprinted from Forssbohm et al. 2008 , with permission, from the European Respiratory Society, © 2008.)
Frequency of bacteriological culture confirmation of Mtb complex among reported tuberculosis cases by site of disease, Germany, 1996–2000
Culture confirmation | |||
---|---|---|---|
Present | Absent | Total | |
Total | 18,501 (70%) | 7801 | 26,302 |
Pulmonary | 15,134 (73%) | 5493 | 20,627 |
Extrapulmonary | 3367 (59%) | 2308 | 5675 |
Lymphatic, peripheral | 859 (51%) | 826 | 1685 |
Lymphatic, intrathoracic | 223 (39%) | 352 | 575 |
Pleural | 579 (55%) | 470 | 1049 |
Genitourinary | 746 (78%) | 212 | 958 |
Osteoarticular | 326 (71%) | 131 | 457 |
Miliary | 126 (69%) | 58 | 184 |
Meningeal | 91 (68%) | 42 | 133 |
Peritoneal | 65 (56%) | 51 | 116 |
Cutaneous | 70 (61%) | 45 | 115 |
Other | 282 (70%) | 121 | 403 |
1 Data modified from Forssbohm et al. 2008.
2 Data are presented as n or n (%).
Lymph Node TB
Intrathoracic lymph node tb.
Intrathoracic lymph node TB is relatively more frequent in children than in adults, usually in association with primary pulmonary TB. It may be noted on the chest radiograph as mediastinal enlargement, sometimes associated with calcification ( Fig. 7 ). The use of CT scanning has shown the relative insensitivity of chest radiographs.

Silicotuberculosis. (Figure reprinted from Fuehner et al. 2007 , with permission, from Springer, © 2007.)
Often, enlarged lymph nodes are present only on one side of the mediastinum, although they may be bilateral. The lymph nodes may become so large that they cause bronchial obstruction and collapse of an adjacent region of the lung. If they perforate into the bronchial tract, this may result in sublobar or lobar pneumonia ( Fig. 8 ). The differential diagnosis includes other infectious etiologies such as nontuberculosis mycobacteria (NTM) and fungal infection, malignancy (lymphoma, lung cancer), sarcoidosis, and silicosis.

Pneumonia of left upper lobe (lingula) caused by lymph node perforation. (Figure provided by Robert Loddenkemper.)
The diagnosis can be established by bronchoscopy ( Chung and Lee 2000 ) ( Fig. 9 ), which may be used also as an adjunctive therapeutic measure by removing obstructing intrabronchial lesions or by placement of an intrabronchial stent ( Lee et al. 2010 ). If hilar or mediastinal intrathoracic lymph node involvement is the sole TB manifestation, transbronchial needle aspiration (“blind” TBNA or under ultrasound guidance as EBUS) or mediastinoscopy may establish the etiology. Rapid molecular diagnostic methods have been successfully applied to EBUS-derived lymph node samples ( Dhasmana et al. 2014 ). This provides the opportunity to confirm clinically suspected disease promptly and also to obtain samples for drug sensitivity testing.

CT of the chest and bronchoscopy showing lymph node perforation, before and after treatment for TB. (Figure provided by Robert Loddenkemper.)
Extrathoracic Lymph Node TB
Extrathoracic lymph node TB is one of the most frequent EPTB manifestations in adults. In Germany, for example, it accounts for 30% of EPTB ( Forssbohm et al. 2008 ). In most cases, the cervical/supraclavicular ( Fig. 10 ), and more rarely the axillary or inguinal, lymph nodes are involved.

Extrathoracic lymph node disease. ( Left figure, provided by the authors; right figure, reprinted from Siemon 2007 , with permission, from Springer, © 2007.)
At first, the lymph nodes are firm, painless, and not warm ( Fig. 10 ), but they may later become fluctuant, matted together, and inflamed. If not treated, they may perforate through the skin and create nonhealing fistulas.
The differential diagnosis includes other infectious etiologies such as NTM and fungal infection, malignancy (lymphoma, [lung] cancer), and sarcoidosis. The diagnosis is established by needle aspiration, occasionally by surgical biopsy.
Tuberculous Pleurisy
Tuberculous pleurisy is one of the most frequent EPTB manifestations. In Germany, it accounts for 19% of EPTB cases ( Forssbohm et al. 2008 ). It is more frequent (15%–90%) in HIV coinfection, being seen in patients with higher blood CD4 counts ( Gopi et al. 2007 ). As with pulmonary TB, and contrary to other forms of EPTB, males are more often affected than females. Usually, it occurs in the young; however, in countries with a low TB incidence, pleural disease may develop at older ages as well.
It can present acutely with high fever or as a subacute/chronic illness. Clinical features may start with pleuritic chest pain (dry pleurisy), but the predominant symptom, caused by pleural effusion, is breathlessness. Physical signs of effusion are dullness on percussion and reduced breath sounds at the affected site. The size of the effusion can vary between very small to almost complete involvement of the hemithorax; it is rarely bilateral ( Fig. 11 ). Pulmonary TB changes are present in the minority, but studies have shown that in up to 20%, Mtb can be found in sputum ( Parimon et al. 2008 ).

Unilateral large left pleural effusion attributable to tuberculosis. (Figure reprinted from Fuehner et al. 2007 with permission, from Springer, © 2007.)
The differential diagnosis includes all causes of pleural effusion. The diagnosis is established by investigation of the pleural fluid and/or by pleural biopsies (closed-needle biopsies or thoracoscopic biopsies [ Light 2010 ]). The diagnosis of pleural TB is hampered by its paucibacillary nature ( Chegou et al. 2008 ). Less than 10% of pleural fluids are smear-positive, and culture is positive in only ∼30% (20%–50%) of cases. However, in HIV-positive patients, the fluid is much more likely to be smear- and culture-positive for Mtb if the CD4 count is <100 cells/μL ( Heydermann et al. 1998 ). The exudative fluid is, in the majority of cases, predominantly lymphocytic, although in ∼10%, mainly polymorphonuclear leukocytes are found ( Bielsa et al. 2013 ).
Several laboratory and microbiological tests have been developed during recent years, including nucleic acid amplification and detection, markers of specific and nonspecific immune response such as adenosine deaminase (ADA), and IGRA. These may help in diagnosing TB as the cause of pleural effusion and have a reasonable high sensitivity and specificity ( Trajman et al. 2008 ; Light 2010 ). However, at least in countries with a low TB prevalence, the accuracy may not be sufficient in individual cases ( Hooper et al. 2009 , 2010 ). The diagnostic yield of closed-needle pleural biopsies, especially under ultrasound guidance, is up to 80% ( Diacon et al. 2003 ; Koegelenberg et al. 2010 ).
The accuracy of medical thoracoscopy (pleuroscopy) is almost 100%, as the pathologist is provided with multiple biopsies selected under direct vision ( Figs. 12 and and13). 13 ). Culture is therefore more often positive (78% in one study) ( Fig. 14 ), which in turn enables drug susceptibility testing to be performed ( Loddenkemper 1998 ; Loddenkemper et al. 2011 ).

Thoracoscopic image of early TB pleural effusion. Following drainage of 800 mL of serous effusion, typical miliary sago-like nodules in all parts of the right parietal pleura can be seen (1) on the diaphragm and (2) the anterior chest wall. (Figure reprinted from Loddenkemper et al. 2011 , with permission, from Thieme, © 2011.)

Thoracoscopic image of established TB pleural effusion. Following drainage of 2000 mL of serous effusion, inflamed and hyperemic visceral and parietal pleura covered with fibrinous patches is evident. The posterior costovertebral region is shown with the diaphragm (1) and the chest wall (2). The lung cannot be visualized as it is fibrin-covered. The fibrinous adhesions pull the diaphragm cranially, producing tenting, and show vascularization at their base (arrows). (Figure reprinted from Loddenkemper et al. 2011 , with permission, from Thieme, © 2011.)

( A ) Combined histological and bacteriological yield (%) of standard techniques in diagnosis of TB-related pleural effusion. ( B ) Cultural yield (%) of standard techniques in diagnosis of TB-related pleural effusion. (Figure reprinted from Loddenkemper et al. 2011 , with permission, from Thieme, © 2011.)
Once again, it cannot be overemphasized that clinicians need to think of TB as a possible cause of pleural effusions—and hence perform appropriate investigations if they wish to make the diagnosis. This is particularly important given that effusions often spontaneously resolve, although the patient is at high risk of recurrence or more widespread disease if they do not receive anti-TB treatment at that stage.
Pleural thickening, trapped lung, and chronic empyema are potential sequelae of tuberculous pleural effusion. However, extensive fibrous pleural changes and tuberculous empyema ( Fig. 15 ) are rare if an early diagnosis is made and appropriate treatment started ( Sahn 2002 ). There are insufficient data to support evidence-based recommendations regarding the use of adjunctive corticosteroids in the treatment of tuberculous pleurisy ( Engel et al. 2007 ; Light 2010 ). The early removal of pleural fluid may also reduce the long-term impact of residual pleural fibrosis ( Chung et al. 2008 ; Light 2010 ).

Tuberculous pleural empyema. (Figure reprinted from Siemon 2007 , with permission, from Springer, © 2007.)
Bone/Joints
TB affecting the bones and/or joints is one of the most frequent EPTB manifestations in adults. In Germany, skeletal TB accounts for 8% of EPTB cases ( Forssbohm et al. 2008 ), although in high-incidence countries, it is much more common. It presents usually several (5–7) years after the primary pulmonary infection ( Wallgren 1948 ).
The spine is involved in almost half of skeletal TB cases (Pott’s disease). This starts as inflammation of the intervertebral disk, most often in the lower thoracic and upper lumbar vertebrae, and later involves typically two adjacent vertebrae and the intervertebral disk ( Jain and Dhammi 2007 ). The most common first symptom is slowly progressive, often over weeks and months, back pain with occasionally associated abdominal pain. Local tenderness on palpation may be present. At later stages, kyphoscoliosis or even a gibbus may develop. Spinal cord or nerve root compression with sensory or motor symptoms, even paraplegia, can occur. A paraspinal abscess may develop within the psoas muscle and cause pain in the groin, in particular, on hip flexion. However, paraspinal collections can be seen at any site within the vertebral column ( Fig. 16 ). Modern imaging techniques such as CT, MRI, and bone scan have considerably enhanced the diagnostic work up. Whole-spine imaging is recommended as multiple vertebral sites are involved in up to 50% of cases ( Fig. 17 ). The differential diagnosis includes other infectious etiologies such as chronic bacterial infection, NTM, and malignancy (lymphoma, [lung] cancer). The diagnosis is established by open surgical or needle biopsy for both culture and cytology/histology. Surgical treatment may be indicated (e.g., using external fixation) if there is clear vertebral collapse or destruction or increasing pain suggesting imminent risk of spinal cord or nerve root compression ( Fig. 18 ).

Coronal CT image of young male with back pain, fever, and weight loss with tuberculous paraspinal abscess secondary to vertebral involvement. (Figure provided by Marc Lipman.)

Spinal tuberculosis. Whole-spine MRI showing abnormal signal at multiple vertebral levels within cervical, thoracic, and lumbar spine, plus anterior paraspinal collections at sites of disease. (Figure provided by Marc Lipman.)

Spinal tuberculosis. ( A ) Destruction of vertebrae after tuberculous spondylitis; ( B ) treatment with external fixator. (Figure reprinted from Siemon 2007 , with permission, from Springer, © 2007.)
In other bones and joints, the metaphyses of long bones are the most common sites of TB, but hips, knees, shoulders, elbows ( Fig. 19 ), ankles, wrists, or any other bone or joint may be involved ( Watts and Lifeso 1996 ). The diagnosis is established by open surgical or needle biopsy with culture and histology.

Tuberculosis of the elbow joint. Bone loss is present at the distal humerus, its epidondyles, and also the olecranon and coronoidal process of the ulna. (Figure reprinted from Siemon 2007 , with permission, from Springer, © 2007.)
Genitourinary System
Genitourinary system disease includes TB of the kidney, ureter, bladder, and the male and female genital tract ( Cek et al. 2005 ; Kulchavenya 2013 ). In Germany, it is a frequent extrapulmonary manifestation in adults and accounts for 17% of such cases ( Forssbohm et al. 2008 ). This is in marked contrast to the United Kingdom, where it is responsible for ∼3% ( Public Health England 2014 ). A variety of local symptoms can develop depending on the involved organ, usually many years after the primary infection. Systemic symptoms are less common. Many patients are asymptomatic, and TB may only be detected by urinalysis of pyuria with no apparent bacterial growth. This occurs in >90% of cases. Around 50% will have evidence of previous or active TB present on the chest radiograph ( Wilberschied et al. 1999 ).
Urological TB can cause destruction of the involved kidney, usually unilateral, perinephric or psoas abscess, and/or may lead to stenosis of the ureter and/or involvement of the bladder with cystitis, often accompanied by hematuria.
TB of the female genital system presents as tubal or endometrial involvement (endometritis) causing pelvic pain and/or menorrhagia and may cause infertility. The ovaries (adnexitis) can be involved also, and this may mimic an ovarian cancer ( Fig. 20 ). The diagnosis is established by image-guided needle biopsy, laparoscopy, or open surgical biopsy. TB of the vulva is uncommon.

Coronal MRI showing TB of the left ovary (black arrow from caudal). Right ovary (white arrow from lateral) with cystic enlarged follicles. (Figure reprinted from Siemon 2007 , with permission, from Springer, © 2007.)
TB of the male genital system presents mainly as involvement of the scrotum (epididymitis, orchitis), but the prostate may be affected as well. The diagnosis is established by image-guided needle biopsy or open surgical biopsy.
In some patients, mostly caused by late diagnosis, medical treatment may not result in the resolution of symptoms. Thus, additional surgical intervention and reconstruction may be indicated ( Kulchavenya 2013 ).
Abdominal TB includes involvement of the peritoneum with ascites or any intra-abdominal organ ( Tan et al. 2009 ). This has become rare in low-incidence countries; in Germany, it accounts for 2% of EPTB cases ( Forssbohm et al. 2008 ). Although elsewhere, such as the Indian subcontinent and countries (e.g., the United Kingdom) that see TB relatively frequently in these populations, it is more common. Infection can result from swallowing sputum or nonpasteurized milk ( Mycobacterium bovis ) or by hematogenous spread. In the gut, TB may occur in any location from the mouth to the anus, although is most often in the ileum and cecum.
Abdominal pain occurs frequently, sometimes mimicking acute appendicitis. Other presentations include small bowel obstruction or gastrointestinal bleeding. The liver, spleen ( Fig. 21 ), pancreas, and adrenal glands may all be involved. In the latter case, the presentation can be one of insidious adrenal insufficiency, in which patients report lethargy or are noted to have metabolic derangements on blood testing.

Splenic tuberculomas. (Figure reprinted from Siemon 2007 , with permission, from Springer, © 2007.)
The differential diagnosis ( Khan et al. 2006 ) depends on the organs involved and includes other infectious etiologies, malignancy ( Fig. 22 ), and inflammatory bowel disease ( Almadi et al. 2009 ). Tuberculous peritonitis with ascites results in abdominal swelling often accompanied by acute symptoms of fever, anorexia, and weight loss. The final diagnosis is established by investigation of the ascitic fluid, which apart from cytology and mycobacterial smear, molecular diagnostics, and culture, may be tested for adenosine deaminase ( Shen et al. 2013 ) and using IGRA ( Chou et al. 2011 ). Laparoscopy, open surgical biopsy, or colonoscopy ( Sanai and Bzeizi 2005 ) may need to be performed, and the diagnosis of TB of other abdominal organs is often made following surgical biopsy or tissue sampling guided by ultrasound or CT.

Abdominal TB attributable to TB. Pain and weight loss in an Indian male initially considered to result from disseminated abdominal malignancy. CT abdomen: ( A ) Pretreatment peritoneal and mesenteric thickening leading to omental “cake” (note oral contrast given); ( B ) posttreatment normal peritoneum and mesentery (no oral contrast given). (Figure provided by Marc Lipman.)
Central Nervous System
TB of the central nervous system (CNS) includes tuberculous meningitis (TBM) (the most common manifestation) and encephalitis with or without cerebral tuberculomas ( Fig. 23 ). CNS disease is often seen as part of a disseminated illness, with radiological evidence of, for example, miliary TB on chest imaging (see below). It has become rare in low-TB-incidence countries, and in Germany, it accounts for 2% of EPTB cases in adults ( Forssbohm et al. 2008 ). Headache is the predominant local symptom. Others include a decreased level of consciousness, seizures, neck stiffness, vomiting, and those attributable to involvement of cranial nerves. Spinal meningitis with involvement of the peripheral nerves and even paraplegia can occur ( Thwaites et al. 2009 ). The severity of TBM at presentation is strongly associated with prognosis. Various factors and scoring models have been developed to predict the outcomes of TBM, and these are used in the evaluation of different treatment regimens (Chou et al. 2010).

CNS tuberculoma. MRI brain with contrast shows enhancing lesion with associated edema and mass effect in a Somali female presenting with headache and a seizure. Biopsy-cultured Mtb (Figure provided by Marc Lipman.)
Severe complications include cerebral and spinal cord infarction from granulomatous arteritis, midbrain and the brain stem involvement with loss of automatic, involuntary function, as well as the development of hydrocephalus requiring short- and long-term intervention. The pressure effects of an abscess or granuloma in the CNS may be catastrophic. This should be considered during both investigation and subsequent treatment of patients with “high-risk” lesions in, for example, the brain substance or spinal cord ( Thwaites et al. 2009 ).
The differential diagnosis includes other infectious etiologies of meningitis. The diagnosis is established by lumbar puncture and investigation of the spinal fluid ( Thwaites et al. 2009 ). Highly suspicious is a cerebrospinal fluid (CSF) leucocytosis (predominantly lymphocytes) and raised protein and low-glucose values. The diagnosis is hampered by its paucibacillary nature, but it may be enhanced by simple modification of the Ziehl-Neelsen stain and an ESAT-6 intracellular stain ( Feng et al. 2014 ). The sensitivity is improved using the Xpert MTB/RIF ( Nhu et al. 2014 ). Rapid techniques based on nucleic acid amplification such as polymerase chain reaction (PCR) are more sensitive and specific as they detect specific DNA sequences of the organism ( Thwaites et al. 2004a ; Galimi 2011 ; Nhu et al. 2014 ). CT-guided needle biopsies or open surgery may be needed for the diagnosis of tuberculomas ( DeLance et al. 2013 ). MRI will show generally more lesions than CT ( Bernaerts et al. 2003 ), although in many parts of the world, access to MRI is restricted and, although there is a greater radiation exposure, CT may be preferable if serial imaging is required during follow up.
Fundoscopic examination of the eye can reveal evidence of both anterior and posterior chamber disease ( Gupta et al. 2007 ). Apart from presumed inflammatory responses to mycobacterial antigen (e.g., phlyctenular conjunctivitis, episcleritis, and anterior uveitis), papilloedema may be present in patients with CNS involvement. Choroidal tubercles can be visualized—and are helpful in the diagnosis of disseminated TB ( Fig. 24 ).

Retinal tuberculous lesions. (Figure reprinted from Siemon 2007 , with permission, from Springer, © 2007.)
Apart from specific anti-TB therapy, adjunctive corticosteroids (either dexamethasone or prednisolone) should be given to all patients with TBM, regardless of disease severity and HIV status ( Thwaites et al. 2004b ). Surgical shunting should be considered early in the patient with hydrocephalus and symptoms of raised intracranial pressure. Other neurosurgical operations may be indicated in patients with seizures or brain or spinal cord compression ( DeLance et al. 2013 ).
Disseminated TB can involve any number of organs ( Zumla et al. 2013 ). Hematogenous spread, when it includes the lungs, is often characterized by the radiological feature of a “miliary” pattern on imaging. This was first described in chest radiographs (where the numerous granulomas were felt to resemble millet seed, i.e., 1–4-mm rounded opacities scattered throughout both lungs), although is also applicable to CT imaging ( Fig. 25 ). In Germany, it accounts for 3% of EPTB cases ( Forssbohm et al. 2008 ).

Miliary TB on chest radiograph and CT. (Figure reprinted from Fuehner et al. 2007 , with permission, from Springer, © 2007.)
The differential diagnosis of this radiographic appearance includes other infections (e.g., fungi, nocardia and healed varicella pneumonia) as well as malignancy (in particular, thyroid, breast, and renal cancers, plus melanoma and osteosarcoma) and conditions that result in granulomas) (e.g., sarcoidosis). Hence, clinicians must ensure that a diagnosis of miliary TB is based on as much laboratory information as possible ( Ray et al. 2013 ) and that patients who are started on anti-TB treatment are carefully monitored for response and/or the development of new symptoms and signs. Patients may develop an acute respiratory distress syndrome (ARDS) associated with a high mortality despite intensive care ( Erbes et al. 2006 ; Lee et al. 2011 ). The benefit of adjunctive corticosteroid administration in patients with miliary TB is unclear ( Ray et al. 2013 ).
OTHER TB MANIFESTATIONS
TB can present at any body site. Therefore, clinicians should consider sending samples for mycobacterial molecular analysis, smear, and culture, as well as histo- or cytopathology. This situation occurs most frequently when patients present either with cutaneous disease (typically nonhealing lesions that have been treated for some time with standard antibacterials) ( Fig. 26 ) or to breast, or ear, nose, and throat surgeons. In Germany, other TB manifestations account for 9% of all extrapulmonary TB cases ( Forssbohm et al. 2008 ).

Tracheo-cutaneous fistula with scrofuloderma. (Figure reprinted from Siemon 2007 , with permission, from Springer, © 2007.)
TB pericarditis is a globally important condition with a distinctly poor prognosis if not diagnosed promptly ( Fig. 27 ). It is usually seen as an active acute or subacute condition associated with systemic features plus a pericardial effusion, which may lead to tamponade ( Syed and Mayosi 2007 ). Adjunctive treatment with corticosteroids is recommended ( Evans 2008 ). Constrictive pericarditis secondary to pericardial calcification can also occur ( Fig. 28 ). Here, the initial (primary) infection is thought to have occurred several years earlier and was often asymptomatic. Over time, the patient notices increasing exercise limitation, yet even then may present to health-care services with apparent acute clinical decompensation.

Tuberculous pericarditis with bilateral pleural effusions. ( A ) CT; ( B ) echocardiography. (Figure reprinted from Siemon 2007 , with permission, from Springer, © 2007.)

Calcification after tuberculous pericarditis. (Figure reprinted from Uehlinger et al. 1959 , with permission, from EMH Schweizer Ärzteverlag, © 1959.)
The calcification associated with TB is generally thick, confluent, and irregular, although these features cannot be relied on. CT or MRI are useful investigations but should not detract from efforts to isolate or detect an organism (which may be from other sites including the lung). This may be unsuccessful (particularly with the chronic presentation). The patient will often have a history of exposure to TB, usually from having spent time as a child in a TB-endemic country. Anti-TB treatment should be given, in addition to cardiac and surgical management as needed ( Syed and Mayosi 2007 ).
TREATMENT OF TB
The diagnosis of active TB (and hence the decision to start anti-TB drug therapy without which the great majority of cases will not be cured) uses information derived from the mycobacteria (e.g., positive AFB smear, molecular diagnostic test or culture), a compatible host response (e.g., cavitation on a chest radiograph), and environmental factors (e.g., a history of contact with TB in a person with consistent clinical features, or acquisition of HIV infection leading to altered [and impaired] immunity).
Prompt initiation of treatment will reduce morbidity and mortality for the affected individual and also break the cycle of onward transmission that sustains TB. However, treatment is not without its complications. This is most evident in the management of HIV-associated TB ( Khan et al. 2010 ; Schutz et al. 2010 ), in which there are considerable drug–drug interactions between antiretrovirals and anti-TB medication, and an increased risk of the intense inflammatory response provoked by concurrent use of these drugs (IRIS) ( Meintjes et al. 2008 ). This means that in practice, the relative timing of introduction of these agents also needs to be factored ( Blanc et al. 2011 ; Török et al. 2011 ).
Drug treatment is dealt with in more detail in other sections of this book. However, some of the key principles are described in brief here. Effective treatment is one that enables the individual to complete therapy and results in a very high cure rate and minimal risk of relapse owing to the original infection. Thus, drug therapy needs to be efficacious and have few adverse events ( Breen et al. 2006 ). Both of these will maximize adherence to treatment regimens. However, factors such as duration of treatment, pill burden, cost of therapy, and even ensuring that the patient can access a sustained and secure supply of medication ( Capstick et al. 2011 ) will impact on treatment effectiveness. Hence, at both a personal and a societal level, high-quality treatment programs must ensure that safe and effective drug supplies are available and that a TB service can provide regular monitoring with facilities that optimize the patient’s ability to adhere to the prescribed regimen.
Therapeutic combinations should, if at all possible, be based on known sensitivity of the organism to the selected drugs ( World Health Organization 2011 ). The increasing prevalence of drug resistance ( World Health Organization 2013 ) and the ease of global travel mean that no longer can clinicians assume that the infecting organism is likely to be drug-sensitive. This emphasizes the importance of obtaining pretreatment samples for rapid molecular diagnostics and culture, both of which can indicate likely drug sensitivity and hence guide early and ongoing treatment ( Moureet al. 2011 ).
In presumed drug-sensitive disease, treatment should commence with a minimum of three effective drugs. In practice, this means a four-drug therapy with a rifamycin plus isoniazid as well as pyrazinamide and ethambutol for the first 2 mo (by which point, drug susceptibility should be known for any positive Mtb cultures). It can never be assumed that prescribing medication equates to the patient actually taking it, and arrangements must be in place to ensure that adequate treatment support and supervision are available for the duration of therapy.
On-treatment monitoring of individual response may use strategies such as directly observed therapy ( Okanurak et al. 2007 ), pill counts, and urine tests, plus measures of patient characteristics that reflect disease alleviation, e.g., weight gain ( Khan et al. 2006 ) and health status ( Kruijshaar et al. 2010 ).
People on treatment for pulmonary TB can be followed with serial sputum cultures. However, in resource-rich environments, individuals often stop producing sputum within a few weeks of starting an effective treatment regimen ( Hales et al. 2013 ). In cases in which there is doubt regarding adherence with medication or possible treatment failure, it is recommended that the patient be evaluated for therapeutic compliance, pharmacodynamics (e.g., is there any possibility of malabsorption?), drug resistance, or an alternative diagnosis.
The importance of close liaison with other experienced clinicians and microbiologists, as well as public health officials within the setting of a multidisciplinary team meeting or through the use of regular cohort reviews ( Anderson et al. 2014 ), cannot be overemphasized. These provide optimal care for the individual and strengthen public health management of TB and are also an excellent method of education and training for all individuals involved in TB care ( Migliori et al. 2012 ).
The diagnosis of TB enables health-care services to provide more than just treatment for TB. HIV testing should be routinely offered—with studies showing that “opt out” testing in TB clinics is a simple method of achieving this ( Roy et al. 2013 ). Screening for other blood-borne viruses such as hepatitis B and C, as well as assessing nutritional status, blood pressure, blood sugar, and encouraging smoking cessation, can also be highlighted during clinical care as part of a package of person-related health management.
Given the generally excellent response to treatment if therapy is started early, there is now little excuse for “missed diagnoses” and “late presentations” of TB. It remains a condition that in many parts of the world is common and hence usually promptly diagnosed. Here, the clinical issues often center on the need to ensure that correct, effective medication is prescribed and adverse events are minimized. However, in environments where TB is less frequently seen, the recognition that it can present in multiple ways and that TB must be considered early (by both the patient and their health-care provider) within the clinical diagnostic algorithm are of paramount importance. This is made more complex by the increasing numbers of immunocompromised individuals (through, e.g., HIV infection and medical treatments) at risk of developing active TB who, by virtue of their altered host response, will often have a modified presentation of TB disease. Strategies to combat the loss of familiarity with TB in low TB burden areas include the use of clinical networks and multidisciplinary and multiprofessional team working, plus on-going, relevant education in at-risk communities, the general public, and health-care workers.
Editors: Stefan H.E. Kaufmann, Eric J. Rubin, and Alimuddin Zumla
Additional Perspectives on Tuberculosis available at www.perspectivesinmedicine.org
- Aït-Khaled N, Alarcón E, Armengol R, Bissell K, Boillot F, Caminero JA, Chiang C-Y, Clevenbergh P, Dlodlo R, Enarson DA, et al. 2010. Management of tuberculosis: A guide to the essentials of good practice. Paris, France , International Union Against Tuberculosis and Lung Disease (The Union) http://www.theunion.org/what-we-do/publications/technical/english/pub_orange-guide_eng.pdf [ Google Scholar ]
- Almadi MA, Ghosh S, Aljebreen AM. 2009. Differentiating intestinal tuberculosis from Crohn’s disease: A diagnostic challenge . Am J Gastroenterol 104 : 1003–1012. [ PubMed ] [ Google Scholar ]
- Anderson C, White J, Abubakar I, Lipman M, Tamne S, Anderson SR, deKoningh J, Dart S. 2014. Raising standards in UK TB control: Introducing cohort review . Thorax 69 : 187–189. [ PubMed ] [ Google Scholar ]
- Bernaerts A, Vanhoenacker FM, Parizel PM, Van Goethem JW, Van Altena R, Laridon A, De Roeck J, Coeman V, De Schepper AM. 2003. Tuberculosis of the central nervous system: Overview of neuroradiological findings . Eur Radiol 13 : 1876–1890. [ PubMed ] [ Google Scholar ]
- Bhat VK, Latha P, Upadhya D, Hegde J. 2009. Clinicopathological review of tubercular laryngitis in 32 cases of pulmonary Kochs . Am J Otolaryngol 30 : 327–330. [ PubMed ] [ Google Scholar ]
- Bielsa S, Palma R, Pardina M, Esquerda A, Light RW, Porcel JM. 2013. Comparison of polymorphonuclear- and lymphocyte-rich tuberculous pleural effusions . Int J Tuberc Lung Dis 17 : 85–89. [ PubMed ] [ Google Scholar ]
- Blanc FX, Sok T, Laureillard D, Borand L, Rekacewicz C, Nerrienet E, Madec Y, Marcy O, Chan S, Prak N, et al. 2011. Earlier versus later start of antiretroviral therapy in HIV-infected adults with tuberculosis . N Engl J Med 365 : 1471–1481. [ PMC free article ] [ PubMed ] [ Google Scholar ]
- Blasi F, Matteelli A. 2013. Indicator condition-guided HIV testing in Europe: A step forward to HIV control . Eur Respir J 42 : 572–575. [ PubMed ] [ Google Scholar ]
- Boehme CC, Nicol MP, Nabeta P, Michael JS, Gotuzzo E, Tahirli R, Tarcela Gler M, Blakemore R, Worodria W, Gray C, et al. 2011. Feasibility, diagnostic accuracy, and effectiveness of decentralised use of the Xpert MTB/RIF test for diagnosis of tuberculosis and multidrug resistance: A multicentre implementation study . Lancet 377 : 1495–1505. [ PMC free article ] [ PubMed ] [ Google Scholar ]
- Borgdorff MW, Nagelkerke NJ, Dye C, Nunn P. 2000. Gender and tuberculosis: A comparison of prevalence surveys with notification data to explore sex differences in case detection . Int J Tuberc Lung Dis 4 : 123–132. [ PubMed ] [ Google Scholar ]
- Breen RAM, Smith CJ, Cropley I, Johnson MA, Lipman MCI. 2005. Does immune reconstitution syndrome promote active tuberculosis in patients receiving HAART? AIDS 19 : 1201–1206. [ PubMed ] [ Google Scholar ]
- Breen RAM, Miller RF, Gorsuch T, Smith CJ, Schwenk A, Holmes W, Ballinger J, Swaden L, Johnson MA, Cropley I, et al. 2006. Adverse events and treatment interruption in tuberculosis patients with and without HIV co-infection . Thorax 61 : 791–794. [ PMC free article ] [ PubMed ] [ Google Scholar ]
- Breen RAM, Leonard O, Perrin FMR, Smith CJ, Bhagani S, Cropley I, Lipman MCI. 2008a. How good are systemic symptoms and blood inflammatory markers in detecting individuals with active tuberculosis? A prospective UK cohort study . Int J Tuberc Lung Dis 12 : 44–49. [ PubMed ] [ Google Scholar ]
- Breen RA, Barry SM, Smith CJ, Shorten RJ, Dilworth JP, Cropley I, McHugh TD, Gillespie SH, Janossy G, Lipman MC. 2008b. Clinical application of a rapid lung-orientated immunoassay in individuals with possible tuberculosis . Thorax 63 : 67–71. [ PubMed ] [ Google Scholar ]
- Brown M, Varia H, Bassett P, Davidson RN, Wall R, Pasvol G. 2007. Prospective study of sputum induction, gastric washing, and bronchoalveolar lavage for the diagnosis of pulmonary tuberculosis in patients who are unable to expectorate . Clin Infect Dis 44 : 1415–1420. [ PubMed ] [ Google Scholar ]
- Cain KP, McCarthy KD, Heilig CM, Monkongdee P, Tasaneeyapan T, Kanara N, Kimerling ME, Chheng P, Thai S, Sar B, et al. 2010. An algorithm for tuberculosis screening and diagnosis in people with HIV . N Engl J Med 362 : 707–716. [ PubMed ] [ Google Scholar ]
- Capstick T, Laycock D, Lipman M. 2011. Treatment interruptions and inconsistent supply of anti-TB drugs in the UK . Int J Tuberc Lung Dis 15 : 754–760. [ PubMed ] [ Google Scholar ]
- Caws M, Thwaites G, Dunstan S, Hawn TR, Lan NT, Thuong NT, Stepniewska K, Huyen MN, Bang ND, Loc TH, et al. 2008. The influence of host and bacterial genotype on the development of disseminated disease with Mycobacterium tuberculosis . PLoS Pathog 4 : e1000034. [ PMC free article ] [ PubMed ] [ Google Scholar ]
- Cek M, Lenk S, Naber KG , Bishop MC, Johansen TE, Botto H, Grabe M, Lobel B, Redorta JP, Tenke P. 2005. Members of the Urinary Tract Infection (UTI) Working Group of the European Association of Urology (EAU) Guidelines Office. EAU guidelines for the management of genitourinary tuberculosis . Eur Urol 48 : 353–362. [ PubMed ] [ Google Scholar ]
- Chegou NN, Walzl G, Bolliger CT, Diacon AH, van den Heuvel MM. 2008. Evaluation of adapted whole-blood interferon-γ release assays for the diagnosis of pleural tuberculosis . Respiration 76 : 131–138. [ PubMed ] [ Google Scholar ]
- Chou CH, Lin GM, Ku CH, Chang FY. 2001. Comparison of the APACHE II, GCS and MRC scores in predicting outcomes in patients with tuberculous meningitis . Int J Tuberc Lung Dis 14 : 86–92. [ PubMed ] [ Google Scholar ]
- Chou OH, Park KH, Park SJ, Kim SM, Park SY, Moon SM, Chong YP, Kim MN, Lee SO, Choi SH, et al. 2011. Rapid diagnosis of tuberculous peritonitis by T cell-based assays on peripheral blood and peritoneal fluid mononuclear cells . J Infect 62 : 462–471. [ PubMed ] [ Google Scholar ]
- Chowdhury AM, Bhuiya A, Chowdhury ME, Rasheed S, Hussain Z, Chen LC. 2013. The Bangladesh paradox: Exceptional health achievement despite economic poverty . Lancet 382 : 1734–1745. [ PubMed ] [ Google Scholar ]
- Chung HS, Lee JH. 2000. Bronchoscopic assessment of the evolution of endobronchial tuberculosis . Chest 117 : 385–392. [ PubMed ] [ Google Scholar ]
- Chung CL, Chen CH, Yeh CY, Sheu JR, Chang SC. 2008. Early effective drainage in the treatment of loculated tuberculosis pleurisy . Eur Respir J 31 : 1261–1267. [ PubMed ] [ Google Scholar ]
- Courtwright A, Turner AN. 2010. Tuberculosis and stigmatization: Pathways and interventions . Public Health Rep 125 : 434–442. [ PMC free article ] [ PubMed ] [ Google Scholar ]
- Craig SE, Bettinson H, Sabin CA, Gillespie SH, Lipman MCI. 2009. Think TB! Is the diagnosis of pulmonary tuberculosis delayed by antibiotics? Int J Tuberc Lung Dis 13 : 208–213. [ PubMed ] [ Google Scholar ]
- Crump JA, Ramadhani HO, Morrissey AB, Saganda W, Mwako MS, Yang LY, Chow SC, Njau BN, Mushi GS, Maro VP, et al. 2012. Bacteremic disseminated tuberculosis in sub-Saharan Africa: A prospective cohort study . Clin Infect Dis 55 : 242–250. [ PMC free article ] [ PubMed ] [ Google Scholar ]
- DeLance AR, Safaee M, Oh MC, Clark AJ, Kaur G, Sun MZ, Bollen AW, Phillips JJ, Parsa AT. 2013. Tuberculoma of the central nervous system . J Clin Neurosci 20 : 1333–1341. [ PubMed ] [ Google Scholar ]
- de la Rua-Domenech R. 2006. Human Mycobacterium bovis infection in the United Kingdom: Incidence, risks, control measures and review of the zoonotic aspects of bovine tuberculosis . Tuberculosis (Edinb) 86 : 77–109. [ PubMed ] [ Google Scholar ]
- Dhasmana DJ, Ross C, Bradley CJ, Connell DW, George PM, Singanayagam A, Jepson A, Craig C, Wright C, Molyneaux PL, et al. 2014. Performance of Xpert MTB/RIF in the diagnosis of tuberculous mediastinal lymphadenopathy by endobronchial ultrasound . Ann Am Thorac Soc 11 : 392–396. [ PMC free article ] [ PubMed ] [ Google Scholar ]
- Dhillon J, Fourie PB, Mitchison DA. 2014. Persister populations of Mycobacterium tuberculosis in sputum that grow in liquid but not on solid culture media . J Antimicrob Chemother 69 : 437–440. [ PubMed ] [ Google Scholar ]
- Diacon AH, Van de Wal BW, Wyser C, Smedema JP, Bezuidenhout J, Bolliger CT, Walzl G. 2003. Diagnostic tools in tuberculous pleurisy: A direct comparative study . Eur Respir J 22 : 589–591. [ PubMed ] [ Google Scholar ]
- Diel R, Loddenkemper R, Zellweger JP, Sotgiu G, D’Ambrosio L, Centis R, van der Werf MJ, Dara M, Detjen A, Gondrie P, et al. 2013. Old ideas to innovate TB control: Preventive treatment to achieve elimination . Eur Respir J 42 : 785–801. [ PubMed ] [ Google Scholar ]
- Dooley SW Jr, Castro KG, Hutton MD, Mullan RJ, Polder JA, Snider DE Jr. 1990. Guidelines for preventing the transmission of tuberculosis in health-care settings, with special focus on HIV-related issues . MMWR Recomm Rep 39 : 1–29. [ PubMed ] [ Google Scholar ]
- Elliott AM, Hayes RJ, Halwiindi B, Luo N, Tembo G, Pobee JO, Nunn PP, McAdam KP. 1993. The impact of HIV on infectiousness of pulmonary tuberculosis: A community study in Zambia . AIDS 7 : 981–987. [ PubMed ] [ Google Scholar ]
- Engel ME, Matchaba PT, Volmink J. 2007. Corticosteroids for tuberculous pleurisy . Cochrane Database Syst Rev 17 : CD001876. [ PubMed ] [ Google Scholar ]
- Erbes R, Oettel K, Raffenberg M, Mauch H, Schmidt-Ioanas M, Lode H. 2006. Characteristics and outcome of patients with active pulmonary tuberculosis requiring intensive care . Eur Respir J 27 : 1223–1228. [ PubMed ] [ Google Scholar ]
- Evans DJ. 2008. The use of adjunctive corticosteroids in the treatment of pericardial, pleural and meningeal tuberculosis: Do they improve outcome? Respir Med 102 : 793–800. [ PubMed ] [ Google Scholar ]
- Fernando SL, Saunders BM, Sluyter R, Skarratt KK, Goldberg H, Marks GB, Wiley JS, Britton WJ. 2007. A polymorphism in the P2X7 gene increases susceptibility to extrapulmonary tuberculosis . Am J Respir Crit Care Med 175 : 360–366. [ PubMed ] [ Google Scholar ]
- Feng GD, Shi M, Ma L, Chen P, Wang BJ, Zhang M, Chang XL, Su XC, Yang YN, Fan XH, et al. 2014. Diagnostic accuracy of intracellular Mycobacterium tuberculosis detection for tuberculous meningitis . Am J Respir Crit Care Med 189 : 475–481. [ PMC free article ] [ PubMed ] [ Google Scholar ]
- Forssbohm M. 2004. Studie des DZK zur Epidemiologie der Tuberkulose. Abschlussbericht 1996–2000 (22.333 Fälle) [Study of the German Central Committee against Tuberculosis on the epidemiology of tuberculosis. Final report 1996-2000 (22.333 cases) ]. In 28. Informationsbericht des DZK (ed. Loddenkemper R), pp. 66–78. DZK, Berlin. [ Google Scholar ]
- Forssbohm M, Zwahlen M, Loddenkemper R, Rieder HL. 2008. Demographic characteristics of patients with extrapulmonary tuberculosis in Germany . Eur Respir J 31 : 99–105. [ PubMed ] [ Google Scholar ]
- Fuehner T, Stoll M, Bange FC, Welte T, Pletz MW. 2007. Klinik der Lungentuberkulose . Pneumologe 4 : 151–162. [ Google Scholar ]
- Galimi R. 2011. Extrapulmonary tuberculosis: Tuberculous meningitis new developments . Eur Rev Med Pharmacol Sci 15 : 365–386. [ PubMed ] [ Google Scholar ]
- Gopi A, Madhavan SM, Sharma SK, Sahn SA. 2007. Diagnosis and treatment of tuberculous pleural effusion in 2006 . Chest 131 : 880–889. [ PubMed ] [ Google Scholar ]
- Gupta V, Gupta A, Rao NA. 2007. Intraocular tuberculosis—An update . Surv Ophthalmol 52 : 561–587. [ PubMed ] [ Google Scholar ]
- Hales CM, Heilig CM, Chaisson R, Leung CC, Chang KC, Goldberg SV, Gordin F, Johnson JL, Muzanyi G, Saukkonen J, et al. 2013. The association between symptoms and microbiologically defined response to tuberculosis treatment . Ann Am Thorac Soc 10 : 18–25. [ PMC free article ] [ PubMed ] [ Google Scholar ]
- Heyderman RS, Makunike R, Muza T, Odwee M, Kadzirange G, Manyemba J, Muchedzi C, Ndemera B, Gomo ZA, Gwanzura LK, et al. 1998. Pleural tuberculosis in Harare, Zimbabwe: The relationship between human immunodeficiency virus, CD4 lymphocyte count, granuloma formation and disseminated disease . Trop Med Int Health 3 : 14–20. [ PubMed ] [ Google Scholar ]
- Honeyborne I, McHugh TD, Phillips PJ, Bannoo S, Bateson A, Carroll N, Perrin FM, Ronacher K, Wright L, van Helden PD, et al. 2011. Molecular bacterial load assay, a culture-free biomarker for rapid and accurate quantification of sputum Mycobacterium tuberculosis bacillary load during treatment . J Clin Microbiol 49 : 3905–3911. [ PMC free article ] [ PubMed ] [ Google Scholar ]
- Hooper CE, Lee YC, Maskell NA. 2009. Interferon-γ release assays for the diagnosis of TB pleural effusions: Hype or real hope? Curr Opin Pulm Med 15 : 358–365. [ PubMed ] [ Google Scholar ]
- Hooper C, Lee YCG, Maskell N, on behalf of the BTS Pleural Guideline Group. 2010. Investigation of a unilateral pleural effusion in adults: British Thoracic Society pleural disease guideline 2010 . Thorax 65 : i4–ii17. [ PubMed ] [ Google Scholar ]
- Horsburgh CR Jr. 2004. Priorities for the treatment of latent tuberculosis infection in the United States . N Engl J Med 350 : 2060–2067. [ PubMed ] [ Google Scholar ]
- Hu HY, Wu CY, Huang N, Chou YJ, Chang YC, Chu D. 2014. Increased risk of tuberculosis in patients with end-stage renal disease: A population-based cohort study in Taiwan, a country of high incidence of end-stage renal disease . Epidemiol Infect 142 : 191–199. [ PMC free article ] [ PubMed ] [ Google Scholar ]
- Huang CC, Tchetgen ET, Becerra M, Cohen T, Hughes KC, Zhang Z, Calderon R, Yataco R, Contreras C, Galea J, et al. 2014. The effect of HIV-related immunosuppression on the risk of tuberculosis transmission to household contacts . Clin Infect Dis 58 : 765–774. [ PMC free article ] [ PubMed ] [ Google Scholar ]
- Jain AK, Dhammi IK. 2007. Tuberculosis of the spine: A review . Clin Orthop Relat Res 460 : 39–49. [ PubMed ] [ Google Scholar ]
- Khan A, Sterling TR, Reves R, Vernon A, Horsburgh CR. 2006. Lack of weight gain and relapse risk in a large tuberculosis treatment trial . Am J Respir Crit Care Med 174 : 344–348. [ PubMed ] [ Google Scholar ]
- Khan FA, Minion J, Pai M, Royce S, Burman W, Harries AD, Menzies D. 2010. Treatment of active tuberculosis in HIV-coinfected patients: A systematic review and meta-analysis . Clin Infect Dis 50 : 1288–1299. [ PubMed ] [ Google Scholar ]
- Koegelenberg CF, Bollinger CT, Theron, Walzl G, Wright CA, Louw M, Diacon AH. 2010. A direct comparison of the diagnostic yield of ultrasound-assisted Abrams and Tru-Cut needle biopsies for pleural tuberculosis . Thorax 65 : 857–862. [ PubMed ] [ Google Scholar ]
- Kroot EJ, Hazes JM, Colin EM, Dolhain RJ. 2007. Poncet’s disease: Reactive arthritis accompanying tuberculosis. Two case reports and a review of the literature . Rheumatology (Oxford) 46 : 484–489. [ PubMed ] [ Google Scholar ]
- Kruijshaar ME, Lipman M, Essink-Bot ML, Lozewicz S, Creer D, Dart S, Sadler H, Maguire H, Abubakar I. 2010. Health status of UK patients with active tuberculosis . Int J Tuberc Lung Dis 14 : 296–302. [ PubMed ] [ Google Scholar ]
- Kulchavenya E. 2013. Best practice in the diagnosis and management of urogenital tuberculosis . Ther Adv Urol 5 : 143–151. [ PMC free article ] [ PubMed ] [ Google Scholar ]
- Lamm DL. 1992. Complications of bacillus Calmette-Guerin immunotherapy . Urol Clin North Am 19 : 565–572. [ PubMed ] [ Google Scholar ]
- Lawn SD, Zumla AI. 2011. Tuberculosis . Lancet 378 : 57–72. [ PubMed ] [ Google Scholar ]
- Lawn SD, Kerkhoff AD, Vogt M, Wood R. 2013. HIV-associated tuberculosis: Relationship between disease severity and the sensitivity of new sputum-based and urine-based diagnostic assays . BMC Med 11 : 231. [ PMC free article ] [ PubMed ] [ Google Scholar ]
- Lee JY, Yi CA, Kim TS, Kim H, Kim J, Han J, Kwon OJ, Lee KS, Chung MJ. 2010. CT scan features as predictors of patient outcome after bronchial intervention in endobronchial TB . Chest 138 : 380–385. [ PubMed ] [ Google Scholar ]
- Lee K, Kim JH, Lee JH, Lee WY, Park MS, Kim JY, Kim KC, Lee MG, Jung KS, Kim YS, et al. 2011. Acute respiratory distress syndrome caused by miliary tuberculosis: A multicentre survey in South Korea . Int J Tuberc Lung Dis 15 : 1099–1103. [ PubMed ] [ Google Scholar ]
- Light RW. 2010. Update on tuberculous pleural effusion . Respirology 15 : 451–458. [ PubMed ] [ Google Scholar ]
- Loddenkemper R. 1998. Thoracoscopy—State of the art . Eur Respir J 11 : 213–221. [ PubMed ] [ Google Scholar ]
- Loddenkemper, Praveen, Noppen, Pyng. 2011. Medical Thoracoscopy/Pleuroscopy: Manual and Atlas . Georg Thieme, New York. [ Google Scholar ]
- Mase SR, Ramsay A, Ng V, Henry M, Hopewell PC, Cunningham J, Urbanczik R, Perkins MD, Aziz MA, Pai M. 2007. Yield of serial sputum specimen examinations in the diagnosis of pulmonary tuberculosis: A systematic review . Int J Tuberc Lung Dis 11 : 485–495. [ PubMed ] [ Google Scholar ]
- Meintjes G, Lawn SD, Scano F, Maartens G, French MA, Worodria W, Elliott JH, Murdoch D, Wilkinson RJ, Seyler C, et al. 2008. Tuberculosis-associated immune reconstitution inflammatory syndrome: Case definitions for use in resource-limited settings . Lancet Infect Dis 8 : 516–523. [ PMC free article ] [ PubMed ] [ Google Scholar ]
- Menzies D, Fanning A, Yuan L, FitzGerald JM. 2003. Canadian Collaborative Group in Nosocomial Transmission of Tuberculosis. Factors associated with tuberculin conversion in Canadian microbiologiy and pathology workers . Am J Respir Crit Care Med 167 : 599–602. [ PubMed ] [ Google Scholar ]
- Migliori GB, Zellweger JP, Abubakar I, Ibraim E, Caminero JA, De Vries G, D’Ambrosio L, Centis R, Sotgiu G, Menegale O, et al. 2012. European Union standards for tuberculosis care . Eur Respir J 39 : 807–819. [ PMC free article ] [ PubMed ] [ Google Scholar ]
- Millen SJ, Uys PW, Hargrove J, van Helden PD, Williams BG. 2008. The effect of diagnostic delays on the drop-out rate and the total delay to diagnosis of tuberculosis . PLoS ONE 3 : e1933. [ PMC free article ] [ PubMed ] [ Google Scholar ]
- Möller M, de Wit E, Hoal EG. 2010. Past, present and future directions in human genetic susceptibility to tuberculosis . FEMS Immunol Med Microbiol 58 : 3–26. [ PubMed ] [ Google Scholar ]
- Moure R, Muñoz L, Torres M, Santin M, Martín R, Alcaide F. 2011. Rapid detection of Mycobacterium tuberculosis complex and rifampin resistance in smear-negative clinical samples by use of an integrated real-time PCR method . J Clin Microbiol 49 : 1137–1139. [ PMC free article ] [ PubMed ] [ Google Scholar ]
- Navani N, Molyneaux PL, Breen RA, Connell DW, Jepson A, Nankivell M, Brown JM, Morris-Jones S, Ng B, Wickremasinghe M, et al. 2011. Utility of endobronchial ultrasound-guided transbronchial needle aspiration in patients with tuberculous intrathoracic lymphadenopathy: A multicentre study . Thorax 66 : 889–893. [ PMC free article ] [ PubMed ] [ Google Scholar ]
- Nhu NT, Heemskerk D, Thu do DA, Chau TT, Mai NT, Nghia HD, Loc PP, Ha DT, Merson L, Thinh TT, et al. 2014. Evaluation of GeneXpert MTB/RIF for diagnosis of tuberculous meningitis . J Clin Microbiol 52 : 226–233. [ PMC free article ] [ PubMed ] [ Google Scholar ]
- Okanurak K, Kitayaporn D, Wanarangsikul W, Koompong C. 2007. Effectiveness of DOT for tuberculosis treatment outcomes: A prospective cohort study in Bangkok, Thailand . Int J Tuberc Lung Dis 11 : 762–768. [ PubMed ] [ Google Scholar ]
- Parimon T, Spitters CE, Muangman N, Euathrongchit J, Oren E, Narita M. 2008. Unexpected pulmonary involvement in extrapulmonary tuberculosis patients . Chest 134 : 589–594. [ PubMed ] [ Google Scholar ]
- Public Health England. 2014. http://www.hpa.org.uk/Topics/InfectiousDiseases/InfectionsAZ/Tuberculosis/TBUKSurveillanceData/EnhancedTuberculosisSurveillance/nTBEnhanced11site/ (accessed 18 April 2014).
- Ray S, Talukdar A, Kundu S, Khanra D, Sonthalia N. 2013. Diagnosis and management of miliary tuberculosis: Current state and future perspectives . Ther Clin Risk Manag 9 : 9–26. [ PMC free article ] [ PubMed ] [ Google Scholar ] Retracted
- Rice B, Elford J, Yin Z, Kruijshaar M, Abubakar I, Lipman M, Pozniak A, Kall M, Delpech V. 2013. Decreasing incidence of tuberculosis among HIV diagnosed heterosexuals in England and Wales . AIDS 27 : 1151–1157. [ PubMed ] [ Google Scholar ]
- Rieder HL, Watson JM, Raviglione MC, Forssbohm M, Migliori GB, Schwoebel V, Leitch AG, Zellweger JP. 1996. Surveillance of tuberculosis in Europe. Working Group of the World Health Organization (WHO) and the European Region of the International Union Against Tuberculosis and Lung Disease (IUATLD) for uniform reporting on tuberculosis cases . Eur Respir J 9 : 1097–1104. [ PubMed ] [ Google Scholar ]
- Roy A, Anaraki S, Hardelid P, Catchpole M, Rodrigues LC, Lipman M, Perkins S, Roche A, Stagg H, Figueroa J, Abubakar I. 2013. Implementing universal HIV testing in London tuberculosis clinics: A cluster randomised controlled trial . Eur Respir J 41 : 627–634. [ PubMed ] [ Google Scholar ]
- Sahn SA. 2002. Pleural thickening, trapped lung, and chronic empyema as sequelae of tuberculous pleural effusion: Don’t sweat the pleural thickening . Int J Tuberc Lung Dis 6 : 461–464. [ PubMed ] [ Google Scholar ]
- Sanai FM, Bzeizi KI. 2005. Systematic review: Tuberculous peritonitis—Presenting features, diagnostic strategies and treatment . Aliment Pharmacol Ther 22 : 685–700. [ PubMed ] [ Google Scholar ]
- Schoch OD, Rieder P, Tueller C, Altpeter E, Zellweger JP, Rieder HL, Krause M, Thurnheer R. 2007. Diagnostic yield of sputum, induced sputum, and bronchoscopy after radiologic tuberculosis screening . Am J Respir Crit Care Med 175 : 80–86. [ PubMed ] [ Google Scholar ]
- Schutz C, Meintjes G, Almajid F, Wilkinson RJ, Pozniak A. 2010. Clinical management of tuberculosis and HIV-1 co-infection . Eur Respir J 36 : 1460–1481. [ PubMed ] [ Google Scholar ]
- Shen YC, Wang T, Chen L, Yang T, Wan C, Hu QJ, Wen FQ. 2013. Diagnostic accuracy of adenosine deaminase for tuberculous peritonitis: A meta-analysis . Arch Med Sci 9 : 601–607. [ PMC free article ] [ PubMed ] [ Google Scholar ]
- Siemon G. 2007. Extrapulmonale tuberkulose . Pneumolog 4 : 163–174. [ Google Scholar ]
- Syed FF, Mayosi BM. 2007. A modern approach to tuberculous pericarditis . Prog Cardiovasc Dis 50 : 218–236. [ PubMed ] [ Google Scholar ]
- Tan KK, Chen K, Sim R. 2009. The spectrum of abdominal tuberculosis in a developed country: A single institution’s experience over 7 years . J Gastrointest Surg 13 : 142–147. [ PubMed ] [ Google Scholar ]
- TB CARE I. 2014. International standards for tuberculosis care . 3rd ed TB CARE I, The Hague, Netherlands. [ Google Scholar ]
- Thwaites GE, Caws M, Chau TT, Dung NT, Campbell JI, Phu NH, Hien TT, White NJ, Farrar JJ. 2004a. Comparison of conventional bacteriology with nucleic acid amplification (amplified mycobacterium direct test) for diagnosis of tuberculous meningitis before and after inception of antituberculosis chemotherapy . J Clin Microbiol 42 : 996–1002. [ PMC free article ] [ PubMed ] [ Google Scholar ]
- Thwaites GE, Nguyen DB, Nguyen HD, Hoang TQ, Do TT, Nguyen TC, Nguyen QH, Nguyen TT, Nguyen NH, Nguyen TN, et al. 2004b. Dexamethasone for the treatment of tuberculous meningitis in adolescents and adults . N Engl J Med 351 : 1741–1751. [ PubMed ] [ Google Scholar ]
- Thwaites G, Fisher M, Hemingway C, Scott G, Solomon T, Innes J, British Infection Society. 2009. British Infection Society guidelines for the diagnosis and treatment of tuberculosis of the central nervous system in adults and children . J Infect 59 : 167–187. [ PubMed ] [ Google Scholar ]
- Török ME, Yen NT, Chau TT, Mai NT, Phu NH, Mai PP, Dung NT, Chau NV, Bang ND, Tien NA, et al. 2011. Timing of initiation of antiretroviral therapy in human immunodeficiency virus (HIV)—Associated tuberculous meningitis . Clin Infect Dis 52 : 1374–1383. [ PMC free article ] [ PubMed ] [ Google Scholar ]
- Trajman A, Pai M, Dheda K, van Zyl Smit R, Zwerling AA, Joshi R, Kalantri S, Daley P, Menzies D. 2008. Novel tests for diagnosing tuberculous pleural effusion: What works and what does not? Eur Respir J 31 : 1098–1106 [ PubMed ] [ Google Scholar ]
- Uehlinger A, Schaub F, Bühlmann A. 1959. Zur klinik und pathophysiologie der pericarditis constrictiva . Schweiz Med Wochenschr 89 : 853–862. [ PubMed ] [ Google Scholar ]
- Wallgren A. 1948. The time-table of tuberculosis . Tubercle 29 : 245–251. [ PubMed ] [ Google Scholar ]
- Watts HG, Lifeso RM. 1996. Tuberculosis of bones and joints . J Bone Joint Surg Am 78 : 288–298. [ PubMed ] [ Google Scholar ]
- Wilberschied LA, Kaye K, Fujiwara PI, Frieden TR. 1999. Extrapulmonary tuberculosis among foreign-born patients, New York City, 1995 to 1996 . J Immigr Health 1 : 65–75. [ PubMed ] [ Google Scholar ]
- World Health Organization. 2011. Guidelines for the programmatic management of drug-resistant tuberculosis—2011 update , 6th ed. WHO/HTM/TB/2011 WHO, Geneva. [ PubMed ] [ Google Scholar ]
- World Health Organization. 2013. Global tuberculosis report [ Google Scholar ]
- Zumla A, Raviglione M, Hafner R, von Reyn CF. 2013. Tuberculosis . N Engl J Med 368 : 745–755. [ PubMed ] [ Google Scholar ]
Thank you for visiting nature.com. You are using a browser version with limited support for CSS. To obtain the best experience, we recommend you use a more up to date browser (or turn off compatibility mode in Internet Explorer). In the meantime, to ensure continued support, we are displaying the site without styles and JavaScript.
- View all journals
- Explore content
- About the journal
- Publish with us
- Sign up for alerts
- Review Article
- Published: 27 April 2022
Anti-tuberculosis treatment strategies and drug development: challenges and priorities
- Véronique A. Dartois ORCID: orcid.org/0000-0001-9470-5009 1 &
- Eric J. Rubin ORCID: orcid.org/0000-0001-5120-962X 2
Nature Reviews Microbiology volume 20 , pages 685–701 ( 2022 ) Cite this article
45k Accesses
162 Citations
153 Altmetric
Metrics details
- Antimicrobials
- Drug discovery
- Microbiology
- Policy and public health in microbiology
Despite two decades of intensified research to understand and cure tuberculosis disease, biological uncertainties remain and hamper progress. However, owing to collaborative initiatives including academia, the pharmaceutical industry and non-for-profit organizations, the drug candidate pipeline is promising. This exceptional success comes with the inherent challenge of prioritizing multidrug regimens for clinical trials and revamping trial designs to accelerate regimen development and capitalize on drug discovery breakthroughs. Most wanted are markers of progression from latent infection to active pulmonary disease, markers of drug response and predictors of relapse, in vitro tools to uncover synergies that translate clinically and animal models to reliably assess the treatment shortening potential of new regimens. In this Review, we highlight the benefits and challenges of ‘one-size-fits-all’ regimens and treatment duration versus individualized therapy based on disease severity and host and pathogen characteristics, considering scientific and operational perspectives.
Similar content being viewed by others
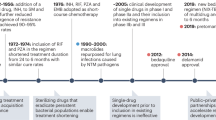
Therapeutic developments for tuberculosis and nontuberculous mycobacterial lung disease
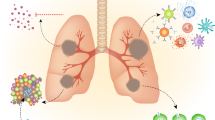
Therapeutic host-directed strategies to improve outcome in tuberculosis
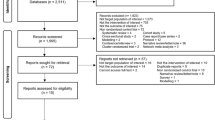
Efficacy of anti-tuberculosis drugs for the treatment of latent tuberculosis infection: a systematic review and network meta-analysis
Introduction.
The Nobel Prize-winning discovery of streptomycin has enabled the treatment of several infectious diseases, including tuberculosis (TB). Since then, many newer antibiotics have been combined into anti-TB drug treatment regimens that have saved millions of lives. However, despite advances in the past, until the emergence of SARS-CoV-2, TB was the leading cause of infectious disease mortality worldwide, with more than 1 million deaths annually.
Although the current standard treatment for TB is effective, it is also unwieldy. Patients with uncomplicated drug-susceptible TB are required to take multiple antibiotics for 6 months. Since compliance is inconsistent, WHO recommends that this be directly supervised, adding an enormous layer of infrastructure to an exceptionally long treatment programme. With the rise of drug resistance, treatment failure rates have increased along with more toxic therapies that are far more costly. Improved interventions could have a substantial effect on our ability to decrease the morbidity and mortality associated with the disease and to limit further spread, as treatment of active TB is the major modality for preventing transmission in most of the world.
We are at an exciting juncture in TB regimen development. For the first time in four decades, a 4-month regimen, containing rifapentine and moxifloxacin, was found non-inferior to the standard 6-month regimen in the treatment of drug-susceptible TB at the 12-month follow-up 1 . In 2019, the first 6-month regimen was approved for the treatment of multidrug-resistant (MDR) and extensively drug-resistant (XDR) TB, comprising only three drugs with two novel mechanisms of action: bedaquiline, pretomanid and linezolid 2 . Yet, shorter, better tolerated and more successful treatments are needed for all patient populations. Accomplishing this will require both new antibiotics and new combinations of approved drugs and clinical candidates. Compared to a decade ago, the anti-TB drug pipeline is in healthy shape with both repurposed and repositioned antibiotic classes as well as drug candidates that act via novel mechanisms of action ( Working Group on New TB Drugs ). Because of this success, the most important research focus has become the prioritization of promising drug regimens. Although there is a considerable amount of active research and development in this area, one major limitation is the lack of validated in vitro and animal models that predict the success of drugs and drug combinations.
Here, we discuss the uneven distribution of TB burden and disease spectrum around the world and its implication on treatment strategies and challenges. We review new technological advances in mycobacteriology, their impact on TB drug discovery and development, and where knowledge gaps remain. Basic and preclinical research priorities are proposed to accelerate the development of curative drug regimens. Not included here are a survey of host-directed therapy approaches, an exhaustive summary of clinical drug candidates and their targets, nor a list of clinical trials of single drugs and drug regimens, all recently and comprehensively reviewed elsewhere 3 , 4 , 5 .
TB burden and spectrum of disease
Burden distribution and inequalities.
TB disease is proceeding asynchronously around the world. In 2019, 30 countries with a high TB burden accounted for 87% of new TB cases and 8 countries accounted for two-thirds of the total cases ( WHO tuberculosis ) 6 . As disease burden is strongly associated with socio-economic conditions 7 , its incidence rates have different trajectories in different parts of the world. In the USA, TB has an incidence similar to amyotrophic lateral sclerosis; in other words, it is a very rare condition. China and the Russian Federation formerly suffered from high TB rates but, although still incurring substantial burden, they are on a better trajectory than most high-burden countries (Fig. 1 ). In other parts of the world, such as in South America, North Africa and parts of Asia, where incidence was moderate a few decades ago, the TB–diabetes syndemic has reverted the trend; diabetes being an important risk factor for developing TB and presenting more complicated disease, higher relapse rates and mortality 8 , 9 . Although several comorbidities can increase susceptibility to TB, HIV-1 is the leading determinant of reactivation risk and thus contributes to the TB burden in sub-Saharan Africa. The long-term repercussions of the COVID-19 pandemic 10 , 11 combined with wealth inequalities will further widen the divide 12 . To a large extent, distinct regions and subcontinents face unique challenges that require tailored improvements in diagnostic and treatment strategies. Yet, treatment guidelines, diagnostics and research needs tend to be defined globally, based on the integration of worldwide statistics.
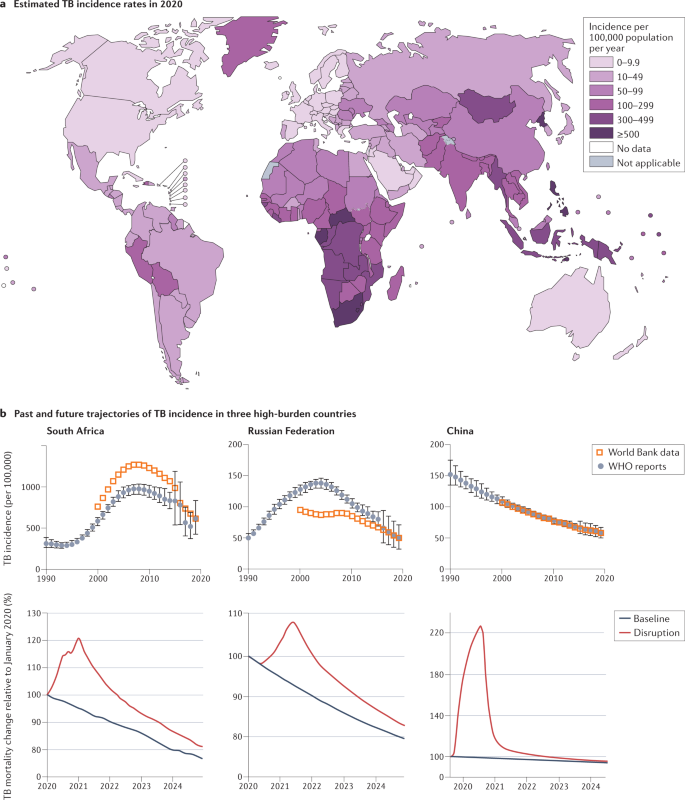
a | Incidence of tuberculosis (TB) per 100,000 population in 2020. Not applicable: WHO criteria for national prevalence survey not met. b | The top graphs represent the incidence of TB in South Africa, the Russian Federation and China from 1990 to 2020. Sub-Saharan Africa has been on an overall trajectory of increased incidence until 2010, mostly driven by the TB–HIV-1 epidemic and the 20-fold increased risk of reactivation in people positive for HIV-1. Initial increase in incidence and mortality in the Russian Federation coincides with the collapse of the Soviet Union and health-care system, which was brought under control after 2000. China has been on a consistent steady decline since 1990. The bottom graphs show the estimated impact of the COVID-19 pandemic on TB mortality in South Africa, the Russian Federation and China up to 2025. Plots were generated using publicly available TB burden data from WHO reports, 5 , 6 and the World Bank database . Part b , top graphs, based on data from WHO global TB reports from 1990 to 2021 and adapted from the World Bank database , CC BY 4.0 ( https://creativecommons.org/licenses/by/4.0/ ). Part a and part b , bottom, adapted with permission from ref. 6 , WHO.
Spectrum of disease
WHO defines latent TB infection (LTBI) as a state of persistent immune response to stimulation by Mycobacterium tuberculosis antigens without evidence of clinically manifested active TB and with bacillary replication absent or below some undefined threshold as a result of immunological control. LTBI affected approximately 1.7 billion individuals in 2014 (ref. 13 ), just under one-quarter of the global population. The rate and risk of LTBI reactivation are greatest within 2 years from infection, then decline from 2 to 5 years and beyond 14 . Quantifying the rates of late reactivation versus early progression is complicated by reinfection in high-incidence areas 15 and difficulties in validating starting assumptions 16 such as the presumption that asymptomatic infection is lifelong 17 . The substantial gaps that remain in our understanding of reactivation hinder our efforts towards TB eradication as the burden of LTBI constitutes an enormous reservoir from which active TB cases can emerge. Identifying and triaging this population will drive our chances to meet the global targets of 90% reduction in TB incidence by 2035 and elimination of TB (less than 1 incident case per 1,000,000 per year) by 2050 (ref. 18 ).
Active pulmonary TB disease either appears within 1–2 years after infection or following reactivation of latent infection in ~5–15% of infected individuals throughout their lifetime 19 . TB disease is defined by progressive bacterial replication and pulmonary necrosis and often but not always includes cavitary lesions that promote the transmission of bacteria 20 . Although the outcome of infection by M. tuberculosis has generally been represented as a bimodal distribution between active and latent TB based on the presence or absence of clinical symptoms, it is now increasingly viewed as a continuous spectrum generated by a varied immunopathology that supports bacterial replication, persistence or killing 21 , 22 , 23 , 24 , extending to within-host heterogeneity 25 .
The current drug regimen used to treat uncomplicated drug-susceptible TB is the outcome of several decades of clinical trials 26 . This short-course therapy with isoniazid, rifampicin, pyrazinamide and ethambutol for 2 months followed by 4 months of isoniazid and rifampicin was implemented four decades ago and has not changed since. By contrast, numerous clinical trials of drug-resistant TB were recently completed or are recruiting, providing a dynamic landscape and opportunities to improve cure rates and reduce therapy duration from 24 to 6 months 2 , 27 . The mandate of major drug discovery initiatives is to develop drugs that, in combination, create shorter, safer and simpler regimens to cure all patients with TB 28 .
The possibility that isoniazid could be used to prevent reactivation of LTBI was considered soon after it was introduced in 1952. Subsequent clinical trials in the 50s and 60s demonstrated a marked reduction in active TB in patients treated with isoniazid and a long-lasting protection 29 , 30 . Based on these results, preventive treatment of TB infection with isoniazid for 6 or 9 months became a widespread approach to TB control and is still in use today. More recently, a rifamycin was added to or substituted for isoniazid, which successfully reduced treatment duration 31 , 32 . Five regimens of various durations that include isoniazid and/or a rifamycin are recommended globally and considered equivalent by measure of effectiveness and hepatotoxicity, though they have not been directly compared in clinical trials 32 , 33 , 34 . Besides historic trials with pyrazinamide 35 , levofloxacin in children 36 and adults 37 , 38 and delamanid (PHOENIx MDR-TB) ( ClinicalTrials.gov , NCT03568383) are currently being tested as prophylactic therapy in household contacts of patients with MDR-TB.
TB infection and disease present as a multifaceted spectrum that can be represented in three dimensions (Fig. 2 ). This multitude of manifestations and microbiological diversity creates formidable treatment challenges (see below). LTBI is now recognized as a dynamic continuum of response to infection 21 , 23 . At the ‘active’ end of the latency spectrum are individuals at high risk of developing active disease (‘progressors’), who would benefit from reactivation risk assessment and treatment. Identifying and curing this population is critical to meet the global targets for TB control and elimination by 2050 (ref. 18 ). The spectrum of LTBI, from true latency to incipient disease, has been comprehensively reviewed elsewhere 20 , 23 . Due to the lack of validated immune and bacteriological markers, LTBI is only operationally defined as a state of persistent immune response to stimulation by M. tuberculosis antigens without evidence of clinically manifested active TB, hampering the triaging of those at greatest risk for progression to TB disease 39 . Overall, basic knowledge gaps are deeper for LTBI than for active disease.
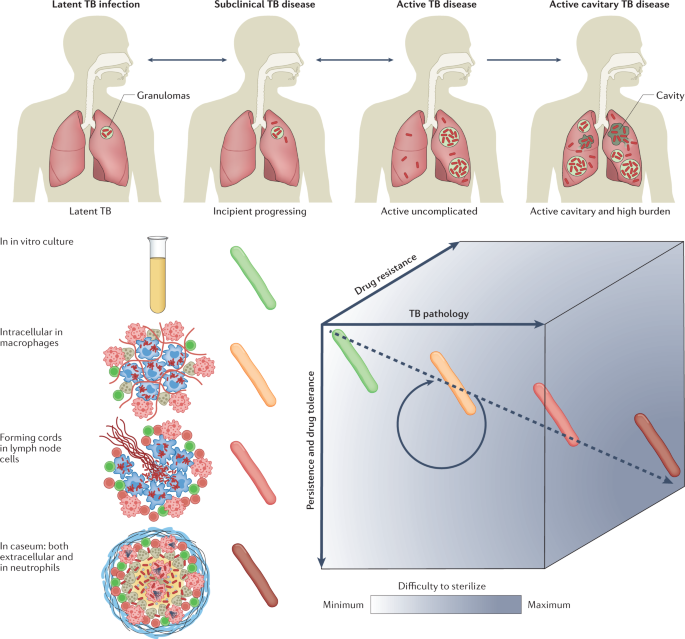
Tuberculosis (TB) presents as a spectrum along three axes: disease pathology and severity, bacterial persistence and drug tolerance, and genetic resistance. The pathology of TB disease is a dynamic continuum from fully latent asymptomatic infection to active disease with high bacterial burden in open cavities, leading to transmission and more frequent treatment failure. Individuals with latent TB infection who are progressing towards incipient TB are at high risk of developing active disease and would benefit from reactivation risk assessment and treatment. The spectrum of immunopathology creates a diversity of microenvironments to which the pathogen responds with metabolic and physiological adaptations leading to drug tolerance or phenotypic drug resistance and persistent disease. Drug tolerance as well as other patient and pathogen factors lead to a spectrum of genetic resistance both in terms of the number of drugs a bacterium is resistant to and the level of resistance to each drug. Such variability along three axes creates a gradient of decreased drug efficacy and lesion sterilization within and across patients, constitutes a multidimensional challenge for health-care programmes and complicates clinical trials.
Active symptomatic TB presents with a range of severity, primarily classified as either cavitary disease or moderate non-cavitary disease. Decades of clinical trials consistently point towards poorer prognostic and treatment outcomes for cavitary disease 40 , 41 , 42 . Recent retrospective analyses have shown that patients with cavities and a high bacterial burden in sputum may require treatment durations of more than 6 months, whereas 4-month therapy was non-inferior to 6-month therapy in patients with non-cavitary minimal disease 43 . The identification of patients who might benefit from shorter treatment durations could therefore only require broadly available chest X-ray radiography and sputum smear methods.
Treatment of drug-susceptible and drug-resistant M. tuberculosis strains requires a minimum of three to four antibiotics in combination, leading to complex patterns of drug susceptibility and resistance. The infecting M. tuberculosis strain is classified as fully drug susceptible, mono-resistant, MDR or XDR. Within the latter two categories, individual drug susceptibility profiles guide the design of patient-tailored drug regimens, which are subject to national guidelines and institutional policies 44 , 45 .
In both LTBI and active disease, host immunity produces diverse microenvironmental niches that support suboptimal growth or complete growth arrest of M. tuberculosis . Many different lesions, with different immune effects, exist simultaneously in a single infected individual. The physiological state of non-replication in bacteria is associated with drug tolerance or phenotypic drug resistance. Indeed, the response to drug treatment is biphasic, with an initial phase of rapid though incomplete clearance of the bulk of infecting bacilli, followed by a longer phase required for complete sterilization of persisting populations 46 . In a defined bacterial population, drug tolerance is either induced by a variety of stresses or is conferred by pre-existing physiological heterogeneity 47 , 48 , 49 , both producing a persister subpopulation . Cell-to-cell variability creates an almost infinite spectrum of phenotypically heterogeneous subpopulations even under uniform conditions 50 , 51 , 52 , 53 , 54 , 55 , which is thought to ensure survival, as at least a small proportion of cells are predisposed to respond to potential threats such as antibiotics, a strategy coined as ‘bet-hedging’ 56 . Cell-to-cell heterogeneity is viewed as the consequence of both stochastic noise and specific regulatory systems that couple cell cycle progression and adaptive bacterial metabolism with changing host-associated environmental stress. Inclusion of adaptive regulatory elements to the stochastic noise mechanism for the generation of phenotypically diverse bacteria within a population has led to a concept referred to as ‘phenomic potential’ 57 . The phenomenon of drug tolerance is particularly acute at the sites of TB disease and is a hallmark of the disease as well as a major factor contributing to protracted therapy duration due to long-standing host–pathogen co-evolution 58 and the resulting adaptations of M. tuberculosis in response to host immunity. There is broad consensus that phenotypic drug resistance contributes to individual variability in TB disease persistence 59 , relapse and reactivation. However, large knowledge gaps remain to determine how in vitro observations of these phenomena can be applied to predict clinical outcome. In the following section, we explore the treatment challenges that are associated with disease complexity.
Treatment challenges
Treatment of all forms of active TB disease requires multiple antibiotics administered for several months 60 . Approved first-line, second-line and third-line agents recommended to treat drug susceptible, MDR and XDR TB, respectively, are listed in Supplementary Table 1 . The variability of disease progression, host response and drug resistance phenotypes complicates treatment and drug discovery but also creates opportunities to stratify patient populations and optimize preventive and therapeutic strategies. Because TB is largely a disease that occurs in resource-constrained countries, existing infrastructure only enables moderately complex interventions. This adds substantial operational and implementation challenges to the already daunting research mandate.
Identifying individuals with LTBI at high risk of reactivation
Currently, the WHO recommends preventive treatment for individuals with LTBI who are at high risk of TB reactivation; that is, people living with HIV-1, infants and children under 5 years of age who are household contacts of patients with pulmonary TB, and patients who receive immunosuppressive therapy 32 , 33 , 34 , 39 . Scientific breakthroughs have recently made it possible to identify patients at risk of reactivating LTBI based on immunobiology rather than on the mainly operational criteria described above 61 . These include non-invasive positron emission tomography–computed tomography (PET–CT) and PET–magnetic resonance imaging (PET–MRI), immune response markers identified by transcriptomics 62 and other omics approaches, genome-wide association studies of host and pathogen, epigenetics, and the identification of differentially expressed genes in individuals who control LTBI and progressors in both patients and animal models (Supplementary Table 2 ). However, validation studies are needed to determine the utility of biomarkers in estimating the size of mycobacterial burden, and markers of protective immunity as surrogates of incipient TB 63 . Although some of these approaches are not compatible with the capacities in resource-poor countries, they critically contribute to our knowledge of reactivation and can form the basis for the development of surrogate markers adapted to the global health realities where TB burdens are highest. Once predictive markers are validated in prospective clinical studies, implementation hurdles will remain to translate ‘high risk’ determination into appropriate treatment of potential progressors 64 . This is further compounded by the caveats of current preventive therapy: the lack of a universal regimen active against drug-resistant M. tuberculosis 32 , the hepatotoxicity 65 and limited sterilizing activity of isoniazid 66 , drug–drug interactions between rifamycins and anti-retroviral therapy 67 , and poor acceptance and completion rates globally 68 . Moreover, adding LTBI therapy to current TB treatment programmes will be a logistical challenge. However, the CDC does currently recommend treatment for every infected individual regardless of the risk of reactivation. The preferred regimens are identical to those recommended by WHO: three rifamycin-based regimens and two alternative monotherapy regimens with daily isoniazid, unless the infecting M. tuberculosis strain is presumptively resistant to both isoniazid and rifampin 33 . This treatment approach is manageable within the USA and, perhaps, other countries with either low or moderate prevalence of LTBI.
Assessing disease severity of patients with active TB
Cavitation status and bacterial burden in sputum are consistently emerging as correlates of disease outcome 42 , 43 . According to the current American Thoracic Society/CDC/Infectious Diseases Society of America Clinical Practice Guidelines 69 , therapy for 9 months is recommended to treat extensive cavitary TB as well as for patients with positive sputum cultures persisting at 2 months. Extended treatment duration is also considered for patients with low body mass index, smokers, individual with diabetes, and individuals with HIV-1. Recently, an in-depth analysis of clinical trial data has revealed that patients with non-cavitary TB, infected with drug-susceptible M. tuberculosis and with a low sputum burden could successfully complete therapy within 4 rather than 6 months. This opens up the possibility for a stratified approach that would tailor treatment duration based on initial disease severity (high bacterial burden in sputum and cavitary disease) as an alternative to the standard 6-month duration for treatment 43 . Shortening treatment when appropriate would improve completion rates and provide welcome relief to health-care systems. Markers such as presence of cavities and bacterial load in sputum can be assessed in resource-limited settings and could be rapidly validated if their correlation with treatment duration were systematically integrated in clinical trials 70 . Pending formal validation, the remaining challenge is the implementation of new flexible treatment guidelines on a global scale given burden inequalities and unique treatment challenges in distinct regions and subcontinents.
Understanding drug tolerance
Targeting drug-tolerant bacterial populations and persisters is the key to achieving faster durable cure 71 . These populations drive the drug recalcitrant nature of both active disease and LTBI. As a research community, we have a good understanding of the microenvironments and stress conditions that exist at the site of disease, but there remain uncertainties about the clinical relevance of each one and how they influence treatment outcome. We have developed assays that reproduce conditions of immune pressure and environment as well as drug-induced stress, in vitro and ex vivo, and we understand how M. tuberculosis adapts to these conditions and how this translates to reduced drug susceptibility in these models (Table 1 ). Ex vivo models, such as those relying on explanted cavity caseum from rabbits 72 and differentially culturable M. tuberculosis retrieved from patient sputum 53 , 73 , most faithfully reproduce in vivo conditions but are inherently resource intensive and mostly suitable to profile approved drugs and clinical development compounds. Drug tolerance of intracellular M. tuberculosis remains a substantial knowledge gap for two reasons. First, a wide range of immortal cell lines and, occasionally, macrophages derived from primary blood or bone marrow monocytes are used for M. tuberculosis infection assays, leading to discrepant potency values. For example, M. tuberculosis is approximately 100-fold less susceptible to rifampicin within hypoxic lipid-loaded macrophages than in normoxic THP-1-derived macrophages 74 , 75 , yet macrophage-like immortalized cell lines are commonly used due to their low cost and ease of maintenance considerations. A comparative review of anti-TB drug potency within infected primary macrophages and common cell lines is lacking. Second, polymorphonuclear leukocytes are increasingly recognized as a crucial and permissive niche of bacterial survival and replication 76 , 77 , 78 , 79 . Rather than eliminating mycobacteria, neutrophils seem to provide a safe haven and transport mycobacteria to macrophages while they retain the potential to damage host tissue 80 . To date, no in vivo or ex vivo assay has been developed to assess the drug tolerance of M. tuberculosis residing within and around neutrophils and other granulocytes. Although the short lifespan of neutrophils may present a challenge, a recent study showed that granulocytes are retained in M. tuberculosis -infected mouse lungs at least as long as monocytes and macrophages, consistent with a model in which M. tuberculosis uses granulocytes as a replicative niche for intracellular growth 81 .
In vivo, we have optimized a wide range of animal models, none fully reproducing human TB, but each with features justifying its utility (Supplementary Table 3 ). It has been suggested that TB should be approached as a polymicrobial infection 82 . Human infection may consist of replicating, dormant (slow-growing or non-growing) and reactivated bacteria in all clinical stages 82 . The relative proportions of these cells may change in response to host immune responses and antibiotic therapy 83 . In other words, the range of phenotypic antibiotic susceptibility in a population is extreme, possibly exceeding that seen for genetic variants. Filling the knowledge gaps to achieve treatment shortening can thus be viewed as a two-step process: first, the inventory of available assays and models that collectively recapitulate micro-environmental conditions encountered in vivo and produce all potentially relevant pathophysiological states of the bacteria, validation of in vitro assay panels that predict clinical treatment shortening, and determination of which animal models are required to bridge in vitro assays and clinical efficacy for new drug candidates; second, the identification of missing assays, models, and bacterial profiles to refine the predictive power of the approach and determination of how they integrate within a patient to drive treatment outcome (that is, the response to treatment may be more complex than the sum of its parts, even if, collectively, assays and models faithfully capture all relevant in vivo conditions). Many have thought that the best way to identify successful therapies is to find agents active in all assay conditions; however, this might well not be true. Prioritizing in vitro assay conditions that have been validated as predictive of in vivo and clinical treatment is likely to yield better drug candidates and help focus resources. Though challenging, all this seems attainable with the ever-faster development of new technologies and a healthy drug pipeline.
Determining high risk of recurrence following therapy completion
Rather than complete bacterial eradication in all treated individuals, apparent bacterial sterilization during and following TB chemotherapy is now understood to include an antibiotic-induced persistent state that forms a relapsing reservoir in a subset of treated patients. Accordingly, ‘high resolution’ determination of the minimum inhibitory concentration (MIC) of isoniazid and rifampicin within the drug-susceptible range (that is, below standard resistance breakpoints ) has shown that higher MIC values were associated with a greater risk of relapse than lower MIC values 84 . We lack validated markers to follow treatment response and distinguish, in real time, patients who need to remain on treatment from hyper-responders who can complete treatment earlier. Identifying patients who might require longer-than-standard treatment duration would minimize costly relapses. A five-gene signature that correlates with the pulmonary inflammatory state, as measured by PET–CT, identified patients at risk of treatment failure 1–4 weeks after start of therapy 85 . More recently, a 22-gene transcriptomic model that predicts cure-associated end-of-therapy was defined for patients infected with drug-susceptible and MDR M. tuberculosis strains 86 . Developing rapid and affordable point-of-care tests that quantify these biomarkers and deploying assays and adapted treatment guidelines are the major unmet needs.
Simplifying and improving treatment of drug-resistant disease
The clinical drug candidate pipeline is healthy, enabling a shift in favour of developing pan-TB or universal drug regimens to treat all forms of drug-susceptible and drug-resistant TB. The success of the NIX-TB trial (NCT02333799), a 6-month three-drug regimen that delivered high cure rates in select patient populations infected with MDR and XDR strains 2 , constitutes a promising step towards this aspirational goal. New diagnostic tools that either simplify or increase the accuracy of drug-resistance profiling are continuously improved to help physicians and health services interpret results and provide faster and more targeted treatment for patients 87 , 88 . Recently, the WHO published a catalogue of 17,000 M. tuberculosis mutations and their association with drug resistance 89 . The report provides a reference standard for the interpretation of mutations conferring resistance to all first-line and a variety of second-line drugs. It complements an elegant tool called SplitStrains , which leverages whole-genome sequencing data to identify and separate mixed M. tuberculosis infections with genetically different strains 90 , a phenomenon that can lead to hetero-resistance and the ensued treatment complications 91 , 92 . The new toolkit enables more precise detection, identification and quantification of multiple infecting strains within a sample. Besides the implementation of new improved diagnostics, the major challenge to eradicate drug-resistant TB is the discovery and development of universal regimens only comprising drugs with novel mechanisms of action and minimum side effects, which would not only be used to treat resistant TB but could also replace the first-line regimen. Towards this goal, the Tuberculosis Drug Accelerator was launched in 2011 as an experiment designed to facilitate collaboration in anti-TB drug discovery by breaking down barriers among competing laboratories and institutions. The Tuberculosis Drug Accelerator is a unique collaboration of academic, pharmaceutical, global non-governmental organizations and governmental organizations that pledge to make medicines affordable to those in need, build knowledge and seek to develop shortened, less toxic, universal drug regimens that can achieve rapid, durable cure irrespective of resistance to existing drugs 28 .
Drug development priorities
The shortcomings of TB treatment can be ascribed to four major challenges: curing TB takes considerably longer than any other bacterial infection of the lungs owing to a combination of drug, pathogen and host factors; drug tolerance fuels and synergizes with drug resistance; single drug and regimen development tested in sequence is inherently slow while tools are emerging to rationally prioritize regimens early in the cascade; and a surprisingly small number of drugs have been tested as preventive therapy of LTBI. Although the division into such broad areas may appear overly schematic, it provides a useful starting point to highlight where future research could focus and identify drug development priorities.
Addressing biological knowledge gaps to optimize treatment
Persistent TB disease is a consequence of immune evasion or the ability of M. tuberculosis to persist and multiply within the very host cells committed to eliminating bacterial pathogens 93 , 94 , suboptimal drug penetration at the sites of disease, and extreme drug tolerance of selected subpopulations, some located in necrotic granulomas and cavity caseum 95 , 96 . Such niche-specific pharmacokinetics and pharmacodynamics present a challenge to ensure optimal drug delivery to bacilli in dynamic physical loci and metabolic states 49 , 97 .
Host-directed approaches that complement antibacterial therapy to accelerate cure generally rely on four major concepts: modulation of pro-inflammatory mediators to dampen inflammation, curb immunopathology and improve lung function and integrity; enhancement of immune and memory response efficacy; enhancement of macrophage and neutrophil bactericidal mechanisms to counter the immune evasion mechanisms of M. tuberculosis ; and disruption of granuloma structure to improve drug penetration and expose the bacilli to drug action. These approaches and corresponding therapies in clinical development are beyond the scope of this Review and have been comprehensively reviewed in recent years 3 , 98 .
A multipronged strategy centred on the pathogen, its metabolic and physiological adaptations, its precise location relative to immune cells and lesion structures, and its susceptibility to drugs and drug combinations in these niches will certainly increase our understanding and might contribute the knowledge required to develop shorter regimens. Recent efforts have focused on the identification of mycobacterial targets that are essential and vulnerable in persistence niches: sputum 99 , 100 , infected mouse lungs, ex vivo and in vitro macrophages, and other relevant environments (reviewed in ref. 97 ). Although host transcriptome analyses are widespread and deliver increasingly robust data, the very low abundance of pathogen transcripts relative to host transcripts in biological samples constitutes a formidable challenge and a limitation of currently available technologies. However, this hurdle is likely to be overcome soon given the ever-improving performance of sequencing platforms and big data analysis. Multi-omics profiling of M. tuberculosis in caseum, foamy hypoxic macrophages, neutrophils and other persister populations in specific lesion compartments will identify pathways and functions that are critical to mycobacterial survival and might represent new antibiotic targets, thus possibly enabling shortening treatment duration. Likewise, a proven CRISPR interference-based functional genomics screen 101 could be applied to persistence environments, such as ex vivo caseum, macrophages and mouse infections, to identify vulnerable targets as well as targets that synergize or antagonize drug treatment 102 .
Leveraging the potential of synergistic drug interactions in multidrug treatment regimens to accelerate durable cure is a growing field that successfully combines experimental, systems biology and computational tools in powerful platforms such as INDIGO and DiaMOND 103 , 104 , 105 , 106 . Collectively, they constitute a living repository of drug interactions on which researchers can build to refine their predictive value. However, although screening for positive and negative drug interactions in vitro is straightforward, understanding which model systems and in vitro assays predict synergies that might translate into the clinic is complex because TB is a polymicrobial disease spread across multiple sites of infection, whereas in vitro synergies are measured in a homogeneous environment using constant drug concentrations. Stochastic and adaptive cell-to-cell bet-hedging leading to a selective advantage for the whole bacterial population is likely to contribute to synergy and antagonism in ways that may be difficult to reproduce in vitro 107 . As a promising example, treatment with bedaquiline in vitro activates a regulatory network that coordinates multiple mechanisms to push M. tuberculosis into a tolerant state, which can be disrupted by knocking out its predicted transcription factors Rv0324 and Rv0880, increasing bedaquiline killing 108 . This finding enabled the prediction that pretomanid could synergize with bedaquiline through inhibition of the Rv0880-response regulon and potentiate killing by bedaquiline at the population level. Although the authors experimentally confirmed the predictions in vitro, identifying bacterial populations to which this applies in animal models and in patients with TB is the critical next step for bedaquiline–pretomanid as the backbone of the highly successful NIX-TB regimen 2 and for all predicted drug–drug synergies in general. In vitro models, such as INDIGO, are pathway based, enabling the formulation of hypotheses to test the underlying biological mechanisms and adjust in vitro growth conditions to improve predictions. Recently, the predictive power of the DiaMOND platform was evaluated using drug combination dose responses measured under eight conditions that reproduce lesion microenvironments. Machine learning was applied to all two-drug and three-drug combinations of ten antibiotics to develop classifiers predictive of multidrug treatment outcome in a mouse model of disease relapse 100 . Identifying positive pharmacodynamics interactions in vitro that translate into the clinic is further complicated by the fluctuating drug concentrations seen by bacilli at the sites of infection as potency interactions inherently depend on the relative concentration of each drug in the combination. To better realize the potential of combination therapy, an interaction landscape is drawn over the full dose–response matrix to delineate synergistic and antagonistic dose regions and quantify the interactions. These can then be mapped back to the in vitro–in vivo correlation to refine in vivo predictions.
Elegant tools exist, and many more are developed, to probe the heterogeneity of bacterial behaviour inside the host niche, survey their metabolic state, and track them during therapy in animal models 49 , 109 , 110 and inpatient bioaerosols 111 . One key pragmatic outcome of these activities is the detection and mapping of M. tuberculosis persisters that survive drug treatment, which would guide prioritization of future research. Validation of the findings from samples of the human lung is challenging although resected lung tissues from patients undergoing elective surgery for drug refractory disease may be an underexploited resource. As mentioned earlier, the contribution of M. tuberculosis bacilli that survive and multiply in and around neutrophils to persistent disease 76 , 77 , 81 has been largely underappreciated, overshadowed by the role of macrophages, which are much more amenable to in vitro and ex vivo studies. However, in sputum and cavity caseum, M. tuberculosis bacilli are primarily extracellular or within neutrophils, with a smaller fraction replicating in macrophages 79 . Consistent with these observations, a signature of active TB is dominated by a neutrophil-driven interferon-inducible gene profile 112 and cavity caseum is often infiltrated with infected neutrophils in both animal models and patients.
Cavitary disease has long been recognized as a predictor of poor treatment outcome and as a factor formally associated with the need for longer treatment duration, especially when associated with positive culture at 2 months 43 , 69 (see above). In a series of new developments, pulmonary PET–CT and μCT imaging have shown that cavities spread M. tuberculosis to other parts of the lungs through the bronchi 113 , 114 , 115 , revealing wide networks of connected lesions with complex morphology, in stark contrast with the oversimplified view that TB granulomas and cavities are isolated spherical structures. The extent of bronchogenic spread, its contribution to disease dissemination, and its impact on treatment outcome in animal models remain to be defined to guide the manipulation of models that better recapitulate this aspect of TB 116 . Thus, targeting treatment not only to cavities but also to airway networks and the bronchial tree might be more important than previously realized to accelerate cure.
Prioritizing promising drug combinations
Owing to a growing pipeline of anti-TB drug candidates (Fig. 3a ), a new paradigm of pan-TB regimen development has gained momentum over the past 5–10 years. New regimens to treat drug-susceptible, MDR and XDR M. tuberculosis infections have become conceivable due to the substantial proportion of drug candidates and recently approved drugs with novel mechanisms of action (Fig. 3b ). Universal regimens would substantially accelerate global efforts to control TB. Given the overwhelming number of potential combinations of three, four and five drugs selected from ten major classes, whether approved or in clinical development, the number one priority is the development of a rationale to predict best performing regimens. So far, the ranking of regimens for clinical trials has been driven by limited preclinical data and could thus be optimized 117 .
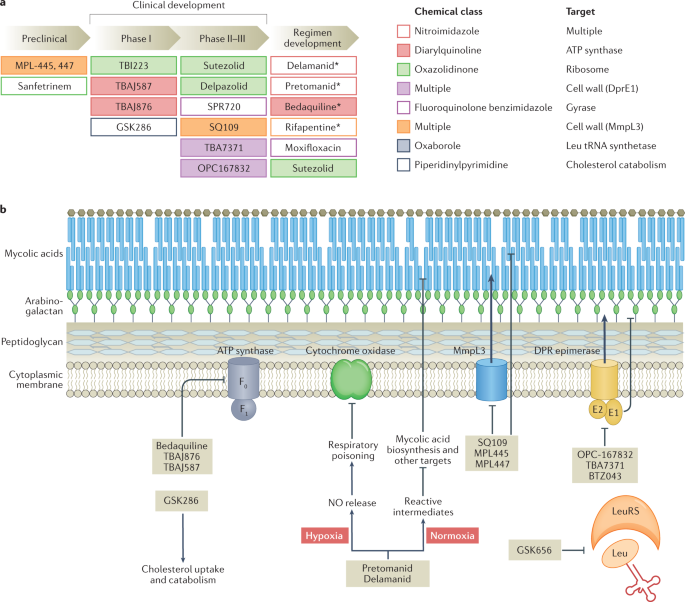
a | Shown are promising drug candidates currently in preclinical and clinical development, including the development of regimens that combine repurposed, repositioned and new drug classes. Approved drugs are indicated by an asterisk (delamanid was approved by the EMA only, and pretomanid was approved by the FDA for use in the bedaquiline–pretomanid–linezolid regimen). Drugs are colour coded by chemical class and target pathway. For a complete list of published candidates currently in the pipeline, from early preclinical development to regulatory approval, and a review of their mechanism of action, see Working Group on New TB Drugs and ref. 183 . b | A simplified version of the cell envelope and the cytoplasmic membrane of Mycobacterium tuberculosis is shown with schematized versions of the targets of recently approved drugs and clinical candidates, with novel mechanisms of action, listed in part a . The majority of novel targets are membrane associated. The diarylquinolines bedaquiline, TBAJ-876 and TBAJ-587 target the ATP synthase. The nitroimidazoles pretomanid and delamanid exhibit a dual mode of action under low and normal oxygen tension, poison multiple essential pathways, and are bactericidal against replicating and non-replicating mycobacteria 184 . SQ109 and the MPL series are the most advanced among a broad panel of agents targeting MmpL3, involved in export of trehalose monomycolate, a mycolic acid component. Three chemically distinct series all target DprE1: OPC167832, TBA7371 and BTZ043 (ref. 185 ). Both MmpL3 and DprE1 are unique to mycobacteria. GSK656 is the first oxaborole in clinical development targeting a mycobacterial tRNA synthetase 186 and GSK286 is a new chemical entity with a novel mechanism of action related to cholesterol catabolism. Part b adapted from ref. 187 , Springer Nature Limited.
Several steps could contribute to a rationale for the prioritization of regimens combining drugs that optimally reach and kill all M. tuberculosis bacilli, thereby maximizing the potential to shorten treatment. We can measure how effectively each drug covers the diverse sites of infection, how much is required to inhibit or kill each mycobacterial subpopulation, and whether adequate concentrations are sustained during a typical dosing interval. Algorithms that integrate drug penetration into major lesion compartments, drug potency against intracellular and extracellular persisters at the sites of disease, host immunity, and efficacy in preclinical models can simulate lesion coverage 118 and prioritize combinations predicted to accelerate sterilization and reduce relapse rates 119 , 120 , 121 , 122 . This approach, combined with predictions of synergistic drug combinations, could deliver an affordable number of promising drug combinations to be tested in resource-intensive animal models that reproduce features of human pathology not seen in mice 123 , 124 , 125 , 126 . Using an iterative strategy, researchers can identify specific features, read-outs and outcomes that predict treatment shortening and further refine the computational tool (Fig. 4 ). Concomitant testing of prioritized regimens in early clinical trials using biomarkers predictive of long-term outcome 127 would therefore enable validation of animal models and refinement of the translational platform. This translational approach is in its infancy but holds promise to spare years of clinical development for new pan-TB drug regimens.
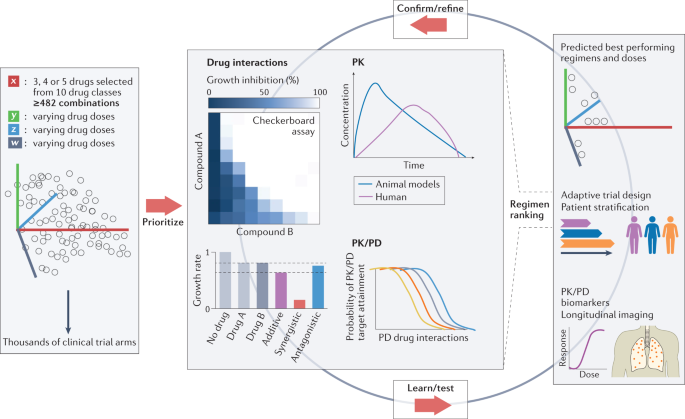
Schematic illustration of the large number of possible combinations if drug candidates selected from 10 drug classes shown in Fig. 3 are combined in 3, 4 or 5 drug regimens (a minimum of 482 combinations assumes only 1 drug per class). In addition, owing to the varying drug doses, varying treatment durations and varying dosing frequencies, the number of clinical trial arms is within the thousands. Resource considerations underline the need for prioritization, using validated in vitro assays, drug interaction platforms, such as INDIGO or DiaMOND, pharmacokinetic (PK) and pharmacodynamic (PD) studies in preclinical species, and translational modelling tools, to select drug combinations with the highest potential to reduce treatment duration and improve cure in patients with tuberculosis, thus reducing the number of clinical trial arms to practical dimensions. New strategies, such as adaptive trial designs, doses and treatment duration tailored to patient characteristics, and longitudinal biomarkers of efficacy are required to accelerate the learning cycle, validate the in vitro and in vivo prioritization tools, and refine the computational approaches. Middle panel, top right, adapted from ref. 188 , Springer Nature Limited. Middle panel, bottom left, adapted from ref. 189 , Springer Nature Limited. Middle panel, top left, reprinted from ref. 190 , Springer Nature Limited.
Conventional assessment of durable cure of active TB in clinical trials is inherently slow and involves very large patient cohorts followed by long follow-up periods. Adaptive trial designs that simplify and accelerate both phase II and phase III 128 have been proposed to evaluate the growing pipeline of drug candidates and leverage the massive efforts dedicated to regimen prioritization using translational platforms that link in silico, in vitro and in vivo data (Supplementary Box 1 ). To maximize the impact of adaptive designs and rapidly identify drug combinations for progression from phase II to phase III, biomarkers predictive of long-term outcome are sorely needed, yet decades of research have not delivered a ‘magic bullet’. Improved bacteriological read-outs (quantitative, longitudinal and continuous measures that are more sensitive to differences than the traditional 8-week sputum conversion end point) have been proposed and are increasingly adopted in clinical trials 129 . Recent efforts have focused on PET–CT to distinguish lesions that resolve rapidly, slowly or not at all in response to conventional versus novel treatment regimens 127 . Key to the success of regimen prioritization, trials should include measurements of drug concentrations to validate the lesion coverage approach for predicting treatment shortening as well as risk stratification algorithms to tailor doses and treatment duration to the extent of disease, immune status, and other validated correlates of poor prognosis 130 . Overall, many innovative and exploratory development strategies remain to be harmonized and formally endorsed to accelerate and enhance our ability to learn.
Breaking the tolerance–resistance link
Bacteria can produce populations of dormant cells that are tolerant to killing by antibiotics. Drug tolerance conferred by slow growth can be genetically encoded or not and sets bacteria on the path to high-level resistance primarily mediated by ‘on-target’ mutations. The discovery of an evolutionary pathway from tolerance to resistance underscores the possibility that treatment strategies that can eliminate tolerant cells to prevent the development of genetic resistance from phenotypically resistant bacterial subpopulations could shorten therapy 131 , 132 . Bacteria can also acquire non-canonical mutations that confer survival advantages in the presence of a given antibiotic, that is, an increased level of tolerance to the drug, likely acting as stepping-stones for acquisition of canonical resistance 133 . In M. tuberculosis , several tolerance mechanisms have been suggested to facilitate the emergence of drug resistance: mistranslation 134 , alteration of propionate metabolism 133 , phase variation in glpK 135 , 136 and hypoxia-induced carbon flux diversion 137 , 138 , 139 . RNase J is disproportionately mutated in drug-resistant clinical M. tuberculosis isolates 133 and preliminary results indicate that deletion of the corresponding gene confers increased tolerance to lethal concentrations of several drugs by reducing their killing rate 140 . Disabling the DosRS dormancy regulon through chemical inhibition by artemisinin suppressed tolerance to isoniazid 141 ; targeting asymmetric cell division leads to the loss of single-cell heterogeneity and faster killing by vancomycin and rifampicin 54 ; and decreasing mistranslation with the aminoglycoside kasugamycin potentiates rifampicin activity in vitro and in mice 142 . Host immune responses may promote drug tolerance through several mechanisms: they apply pressure on bacterial growth, thereby altering drug susceptibility 143 ; they shape the microenvironment to which drugs are exposed at the sites where M. tuberculosis resides and can lead to pH and oxygen concentrations 144 , 145 that may reduce (or occasionally enhance) drug activity; and they alter granuloma histopathology in ways that impact drug access 95 , 146 . Host-directed therapies that target each of these mechanisms have the potential to reduce drug tolerance and resistance 3 . Additional mechanisms of drug tolerance are reviewed elsewhere 147 such as the disproportionate number of toxin–antitoxin systems present in M. tuberculosis that mediate stress-induced adaptation, slowing of growth and drug tolerance. These are early-stage and exploratory observations, yet they lend support to the concept of tackling tolerance to prevent resistance.
Though not falling under the category of tolerance per se, other indirect mechanisms facilitate the emergence of resistance and could be targeted to prevent or limit it. For example, a preliminary study suggests that disruption of the ImuB – DnaN (β-clamp) interaction by griselimycin prevents DnaE2-mediated induced mutagenesis, suggesting that the mycobacterial mutasome might be a good target for novel ‘anti-evolution’ drugs aimed at protecting against emergent resistance 148 ; inhibition of bacterial factors that promote mutagenesis as an anti-evolution strategy 149 ; inhibition of intrabacterial metabolism by promiscuous cytochromes and other enzymes 150 , 151 that may constitute a source of induced resistance; and inhibition of nonspecific drug efflux pumps shown to extrude anti-TB drugs 152 , 153 , 154 .
Preventing reactivation of LTBI
Once a validated biomarker signature of incipient and progressing TB has been established to triage patients with LTBI who would benefit from preventive therapy, TB control programmes will need single drugs or regimens that can be administered safely to individuals with LTBI who are not only at risk of reactivation but are also contacts of individuals who are infected with MDR M. tuberculosis . The five recommended regimens all contain isoniazid and/or a rifamycin and are thus inadequate for these important populations. In addition, individuals with HIV-1 and LTBI should not receive rifampicin if they are treated with antiretroviral agents that are substrates of CYP3A4. More so than for active TB, a universal drug or drug combination is critical as the MDR or XDR status cannot be assessed in LTBI and is only inferred from household contact susceptibility profiling. New pan-TB regimens that successfully shorten treatment of active TB may provide invaluable lessons to tackle persisters in latent TB granulomas and prevent reactivation of LTBI. A ‘sterilizing’ rationale would help design clinical trials with a better potential to cure subclinical, incipient and progressing TB (Box 1 ).
Box 1 Models and trials to test new drugs against reactivation of LTBI
Despite the substantial global population of individuals with subclinical tuberculosis (TB) and the enormous medical need, the knowledge gaps are vast and perhaps more difficult to fill than for active TB due to the nature of latent infection. There is a lack of experimental model systems that fully recapitulate the immune status of the infected individual or Mycobacterium tuberculosis within host physiology 191 , and thus progress has been slower than for active TB. The isoniazid–rifamycin-based recommended regimens were empirically selected for clinical evaluation and have not been compared side-by-side to calibrate model systems. Only observational studies have been completed with individuals who had been in contact with others who are infected with multidrug-resistant (MDR) M. tuberculosis , which suggested that preventive therapy based on second-line drugs may lower the risk of progression to TB disease, and three placebo-controlled trials are ongoing (see the table).
Given the limited breadth of clinical efficacy data available to benchmark latent TB infection (LTBI) in animal models for testing new preventive therapies, one may consider a parallel strategy whereby new drugs or regimens (FDA-approved or EMA-approved, such as levofloxacin and delamanid) are evaluated in clinical trials without prior testing in a preclinical model. If successful, these therapeutic interventions could rapidly translate into the clinic and be integrated into global health policies. Meanwhile, experimental models that accurately recapitulate clinical efficacy, and integrate new technologies that provide quantitative and physiological information about bacteria in lesions, could be validated against existing and new clinical data. Optimizing an animal model that reasonably recapitulates subclinical TB carries many pitfalls 192 . The macaque models control infection in a subset of animals, as seen in human TB, and are likely to be a suitable starting point for a preclinical model to evaluate preventive therapy 25 , 193 . This could go hand in hand with the development of tools to map, track during treatment, and quantify viable bacilli and profile their metabolic status in latent TB granulomas, lymph nodes and other sites of infection.
The repercussions of the COVID-19 outbreak have already made clear that we are unlikely to reach the targets of the WHO ‘end TB strategy’, which call for 80% reduction in TB incidence and 90% reduction of TB deaths by 2030 (ref. 155 ). Even under more favourable circumstances, these goals are often considered an optimistic scenario given the logistical complexities of a 6-month multidrug regimen and the poorer treatment outcomes in the setting of antibiotic-resistant disease. Shorter and more effective therapies could get us a long way towards these millennium goals, but this will take decades if we are to rely on the traditional approach of iterative large clinical trials with small incremental variations in drug regimens. Breakthroughs will come from transformative trial designs and rationally designed regimens with novel mechanisms of action. The success of bedaquiline–pretomanid–linezolid for MDR and XDR M. tuberculosis is one promising step in that direction and has provided a backbone for limitless variations that can be explored. We are hopeful that the preclinical and clinical approaches we described in this Review can lead to the selection and evaluation of drug combinations earlier in the development cascade and greatly accelerate the approval of shorter treatment regimens.
Sociological changes, such as breaking down barriers among collections of academia, non-governmental organizations, funders and drug sponsors, favour collaborative actions that intensify the discovery of breakthrough drug candidates 28 . However, truly accelerating drug development will require changes at all levels, from sustainable funding of basic and applied research through modernized clinical trials. Additionally, ultimately, we might end up with more personalized therapy, which would entail broad changes in how we deliver care in most of the world. For now, big challenges remain but change has already come and there is cause for optimism with a healthy dose of realism.
Dorman, S. E. et al. Four-month rifapentine regimens with or without moxifloxacin for tuberculosis. N. Engl. J. Med. 384 , 1705–1718 (2021).
Article CAS PubMed PubMed Central Google Scholar
Conradie, F. et al. Treatment of highly drug-resistant pulmonary tuberculosis. N. Engl. J. Med. 382 , 893–902 (2020).
Young, C., Walzl, G. & Du Plessis, N. Therapeutic host-directed strategies to improve outcome in tuberculosis. Mucosal Immunol. 13 , 190–204 (2020).
Article CAS PubMed Google Scholar
Perveen, S., Kumari, D., Singh, K. & Sharma, R. Tuberculosis drug discovery: progression and future interventions in the wake of emerging resistance. Eur. J. Med. Chem. 229 , 114066 (2022).
World Health Organization. Global tuberculosis report 2020 (WHO, 2020).
World Health Organization. Global tuberculosis report 2021 (WHO, 2021).
Marais, B. J., Hesseling, A. C. & Cotton, M. F. Poverty and tuberculosis: is it truly a simple inverse linear correlation? Eur. Respir. J. 33 , 943–944 (2009).
Antonio-Arques, V., Franch-Nadal, J. & Cayla, J. A. Diabetes and tuberculosis: a syndemic complicated by COVID-19. Med. Clin. 157 , 288–293 (2021).
Article CAS Google Scholar
Wilkinson, R. J. Tuberculosis and type 2 diabetes mellitus: an inflammatory danger signal in the time of coronavirus disease 2019. Clin. Infect. Dis. 72 , 79–81 (2021).
CAS PubMed Google Scholar
Pai, M., Kasaeva, T. & Swaminathan, S. Covid-19’s devastating effect on tuberculosis care—a path to recovery. N. Engl. J. Med. https://doi.org/10.1056/NEJMp2118145 (2022).
Article PubMed Google Scholar
Glaziou, P. Predicted impact of the COVID-19 pandemic on global tuberculosis deaths in 2020. Preprint at medRxiv https://doi.org/10.1101/2020.04.28.20079582 (2021).
McQuaid, C. F. et al. The potential impact of COVID-19-related disruption on tuberculosis burden. Eur. Respir. J. 56 , 2001718 (2020).
Houben, R. M. & Dodd, P. J. The global burden of latent tuberculosis infection: a re-estimation using mathematical modelling. PLoS Med. 13 , e1002152 (2016).
Article PubMed PubMed Central Google Scholar
Zenner, D., Loutet, M. G., Harris, R., Wilson, S. & Ormerod, L. P. Evaluating 17 years of latent tuberculosis infection screening in north-west England: a retrospective cohort study of reactivation. Eur. Respir. J. 50 , 1602505 (2017).
Dale, K. D. et al. Quantifying the rates of late reactivation tuberculosis: a systematic review. Lancet Infect. Dis. 21 , e303–e317 (2021).
Menzies, N. A. et al. Progression from latent infection to active disease in dynamic tuberculosis transmission models: a systematic review of the validity of modelling assumptions. Lancet Infect. Dis. 18 , e228–e238 (2018).
Behr, M. A., Edelstein, P. H. & Ramakrishnan, L. Is Mycobacterium tuberculosis infection life long? BMJ 367 , l5770 (2019).
Uplekar, M. et al. WHO’s new end TB strategy. Lancet 385 , 1799–1801 (2015).
Kiazyk, S. & Ball, T. B. Latent tuberculosis infection: an overview. Can. Commun. Dis. Rep. 43 , 62–66 (2017).
Pai, M. et al. Tuberculosis. Nat. Rev. Dis. Primers 2 , 16076 (2016).
Barry, C. E. 3rd et al. The spectrum of latent tuberculosis: rethinking the biology and intervention strategies. Nat. Rev. Microbiol. 7 , 845–855 (2009).
Boom, W. H., Schaible, U. E. & Achkar, J. M. The knowns and unknowns of latent Mycobacterium tuberculosis infection. J. Clin. Invest. 131 , e136222 (2021).
Article CAS PubMed Central Google Scholar
Drain, P. K. et al. Incipient and subclinical tuberculosis: a clinical review of early stages and progression of infection. Clin. Microbiol. Rev. 31 , e00021-18 (2018).
Lin, P. L. & Flynn, J. L. The end of the binary era: revisiting the spectrum of tuberculosis. J. Immunol. 201 , 2541–2548 (2018).
Cadena, A. M., Fortune, S. M. & Flynn, J. L. Heterogeneity in tuberculosis. Nat. Rev. Immunol. 17 , 691–702 (2017).
Fox, W., Ellard, G. A. & Mitchison, D. A. Studies on the treatment of tuberculosis undertaken by the British Medical Research Council tuberculosis units, 1946-1986, with relevant subsequent publications. Int. J. Tuberc. Lung Dis. 3 (Suppl. 2), S231–S279 (1999).
Lee, M. et al. Linezolid for treatment of chronic extensively drug-resistant tuberculosis. N. Engl. J. Med. 367 , 1508–1518 (2012).
Aldridge, B. B. et al. The Tuberculosis Drug Accelerator at year 10: what have we learned? Nat. Med. 27 , 1333–1337 (2021).
Glassroth, J., Robins, A. G. & Snider, D. E. Jr Tuberculosis in the 1980s. N. Engl. J. Med. 302 , 1441–1450 (1980).
Debre, R., Perdrizet, S., Lotte, A., Naveau, M. & Lert, F. Isoniazid chemoprophylaxis of latent primary tuberculosis: in five trial centres in France from 1959 to 1969. Int. J. Epidemiol. 2 , 153–160 (1973).
Menzies, D. et al. Four months of rifampin or nine months of isoniazid for latent tuberculosis in adults. N. Engl. J. Med. 379 , 440–453 (2018).
World Health Organization. Latent tuberculosis infection: updated and consolidated guidelines for programmatic management (WHO, 2018).
Sterling, T. R. et al. Guidelines for the treatment of latent tuberculosis infection: recommendations from the National Tuberculosis Controllers Association and CDC, 2020. MMWR Recomm. Rep. 69 , 1–11 (2020).
Ai, J. W., Ruan, Q. L., Liu, Q. H. & Zhang, W. H. Updates on the risk factors for latent tuberculosis reactivation and their managements. Emerg. Microbes Infect. 5 , e10 (2016).
Lobue, P. & Menzies, D. Treatment of latent tuberculosis infection: an update. Respirology 15 , 603–622 (2010).
Seddon, J. A. et al. Levofloxacin versus placebo for the prevention of tuberculosis disease in child contacts of multidrug-resistant tuberculosis: study protocol for a phase III cluster randomised controlled trial (TB-CHAMP). Trials 19 , 693 (2018).
Torre-Cisneros, J. et al. Tuberculosis prophylaxis with levofloxacin in liver transplant patients is associated with a high incidence of tenosynovitis: safety analysis of a multicenter randomized trial. Clin. Infect. Dis. 60 , 1642–1649 (2015).
Fox, G. J. et al. Levofloxacin versus placebo for the treatment of latent tuberculosis among contacts of patients with multidrug-resistant tuberculosis (the VQUIN MDR trial): a protocol for a randomised controlled trial. BMJ Open. 10 , e033945 (2020).
Shah, M. & Dorman, S. E. Latent tuberculosis infection. N. Engl. J. Med. 385 , 2271–2280 (2021).
Aber, V. R. & Nunn, A. J. [Short term chemotherapy of tuberculosis. Factors affecting relapse following short term chemotherapy]. Bull. Int. Union Tuberc. 53 , 276–280 (1978).
Savic, R. M. et al. Defining the optimal dose of rifapentine for pulmonary tuberculosis: exposure-response relations from two phase II clinical trials. Clin. Pharmacol. Ther. 102 , 321–331 (2017).
Franke, M. F. et al. Culture conversion in patients treated with bedaquiline and/or delamanid. A prospective multicountry study. Am. J. Respir. Crit. Care Med. 203 , 111–119 (2021).
Imperial, M. Z. et al. A patient-level pooled analysis of treatment-shortening regimens for drug-susceptible pulmonary tuberculosis. Nat. Med. 24 , 1708–1715 (2018).
Lange, C. et al. Management of drug-resistant tuberculosis. Lancet 394 , 953–966 (2019).
World Health Organization. WHO consolidated guidelines on drug-resistant tuberculosis treatment (WHO, 2019).
Horsburgh, C. R. Jr, Barry, C. E. 3rd & Lange, C. Treatment of tuberculosis. N. Engl. J. Med. 373, (2149–2160 (2015).
Balaban, N. Q. et al. Definitions and guidelines for research on antibiotic persistence. Nat. Rev. Microbiol. 17 , 441–448 (2019).
Gold, B. & Nathan, C. Targeting phenotypically tolerant Mycobacterium tuberculosis . Microbiol. Spectr. https://doi.org/10.1128/microbiolspec.TBTB2-0031-2016 (2017).
Dhar, N., McKinney, J. & Manina, G. Phenotypic heterogeneity in Mycobacterium tuberculosis . Microbiol. Spectr. https://doi.org/10.1128/microbiolspec.TBTB2-0021-2016 (2016).
Aldridge, B. B. et al. Asymmetry and aging of mycobacterial cells lead to variable growth and antibiotic susceptibility. Science 335 , 100–104 (2012).
Wakamoto, Y. et al. Dynamic persistence of antibiotic-stressed mycobacteria. Science 339 , 91–95 (2013).
Manina, G., Griego, A., Singh, L. K., McKinney, J. D. & Dhar, N. Preexisting variation in DNA damage response predicts the fate of single mycobacteria under stress. EMBO J. 38 , e101876 (2019).
Chengalroyen, M. D. et al. Detection and quantification of differentially culturable tubercle bacteria in sputum from patients with tuberculosis. Am. J. Respir. Crit. Care Med. 194 , 1532–1540 (2016).
Rego, E. H., Audette, R. E. & Rubin, E. J. Deletion of a mycobacterial divisome factor collapses single-cell phenotypic heterogeneity. Nature 546 , 153–157 (2017).
Kieser, K. J. & Rubin, E. J. How sisters grow apart: mycobacterial growth and division. Nat. Rev. Microbiol. 12 , 550–562 (2014).
Harms, A., Maisonneuve, E. & Gerdes, K. Mechanisms of bacterial persistence during stress and antibiotic exposure. Science 354 , aaf4268 (2016).
Slayden, R. A., Dawson, C. C. & Cummings, J. E. Toxin-antitoxin systems and regulatory mechanisms in Mycobacterium tuberculosis . Pathog. Dis. 76 , fty039 (2018).
Article Google Scholar
Gagneux, S. Ecology and evolution of Mycobacterium tuberculosis . Nat. Rev. Microbiol. 16 , 202–213 (2018).
Malherbe, S. T. et al. Persisting positron emission tomography lesion activity and Mycobacterium tuberculosis mRNA after tuberculosis cure. Nat. Med. 22 , 1094–1100 (2016).
Sotgiu, G., Centis, R., D’Ambrosio, L. & Migliori, G. B. Tuberculosis treatment and drug regimens. Cold Spring Harb. Perspect. Med. 5 , a017822 (2015).
Barry, C. E. & Mayer-Barber, K. D. Signature required: the transcriptional response to tuberculosis. J. Exp. Med. 218 , e20211665 (2021).
Singhania, A., Wilkinson, R. J., Rodrigue, M., Haldar, P. & O’Garra, A. The value of transcriptomics in advancing knowledge of the immune response and diagnosis in tuberculosis. Nat. Immunol. 19 , 1159–1168 (2018).
Scriba, T. J. et al. Biomarker-guided tuberculosis preventive therapy (CORTIS): a randomised controlled trial. Lancet Infect. Dis. 21 , 354–365 (2021).
Esmail, H., Cobelens, F. & Goletti, D. Transcriptional biomarkers for predicting development of tuberculosis: progress and clinical considerations. Eur. Respir. J. 55 , 1901957 (2020).
Comstock, G. W. & Edwards, P. Q. The competing risks of tuberculosis and hepatitis for adult tuberculin reactors. Am. Rev. Respir. Dis. 111 , 573–577 (1975).
Mitchison, D. A. The action of antituberculosis drugs in short-course chemotherapy. Tubercle 66 , 219–225 (1985).
Ignatius, E. H. & Swindells, S. Are we there yet? Short-course regimens in TB and HIV: from prevention to treatment of latent to XDR TB. Curr. HIV/AIDS Rep. 17 , 589–600 (2020).
Alsdurf, H., Hill, P. C., Matteelli, A., Getahun, H. & Menzies, D. The cascade of care in diagnosis and treatment of latent tuberculosis infection: a systematic review and meta-analysis. Lancet Infect. Dis. 16 , 1269–1278 (2016).
Nahid, P. et al. Executive summary: official American Thoracic Society/Centers for Disease Control and Prevention/Infectious Diseases Society of America clinical practice duidelines: treatment of drug-susceptible tuberculosis. Clin. Infect. Dis. 63 , 853–867 (2016).
Romanowski, K. et al. Predicting tuberculosis relapse in patients treated with the standard 6-month regimen: an individual patient data meta-analysis. Thorax 74 , 291–297 (2019).
Connolly, L. E., Edelstein, P. H. & Ramakrishnan, L. Why is long-term therapy required to cure tuberculosis? PLoS Med. 4 , e120 (2007).
Sarathy, J. P. et al. Extreme drug tolerance of Mycobacterium tuberculosis in caseum. Antimicrob. Agents Chemother. 62 , e02266-17 (2018).
Turapov, O. et al. Phenotypically adapted Mycobacterium tuberculosis populations from sputum are tolerant to first-line drugs. Antimicrob. Agents Chemother. 60 , 2476–2483 (2016).
Daniel, J., Maamar, H., Deb, C., Sirakova, T. D. & Kolattukudy, P. E. Mycobacterium tuberculosis uses host triacylglycerol to accumulate lipid droplets and acquires a dormancy-like phenotype in lipid-loaded macrophages. PLoS Pathog. 7 , e1002093 (2011).
Lakshminarayana, S. B. et al. Comprehensive physicochemical, pharmacokinetic and activity profiling of anti-TB agents. J. Antimicrob. Chemother. 70 , 857–867 (2015).
Kimmey, J. M. et al. Unique role for ATG5 in neutrophil-mediated immunopathology during M. tuberculosis infection. Nature 528 , 565–569 (2015).
Mishra, B. B. et al. Nitric oxide prevents a pathogen-permissive granulocytic inflammation during tuberculosis. Nat. Microbiol. 2 , 17072 (2017).
Dallenga, T. & Schaible, U. E. Neutrophils in tuberculosis — first line of defence or booster of disease and targets for host-directed therapy? Pathog. Dis. 74 , ftw012 (2016).
Eum, S. Y. et al. Neutrophils are the predominant infected phagocytic cells in the airways of patients with active pulmonary TB. Chest 137 , 122–128 (2010).
Parker, H. A., Forrester, L., Kaldor, C. D., Dickerhof, N. & Hampton, M. B. Antimicrobial activity of neutrophils against mycobacteria. Front. Immunol. 12 , 782495 (2021).
Lovewell, R. R., Baer, C. E., Mishra, B. B., Smith, C. M. & Sassetti, C. M. Granulocytes act as a niche for Mycobacterium tuberculosis growth. Mucosal Immunol. 14 , 229–241 (2021).
Evangelopoulos, D. & McHugh, T. D. Improving the tuberculosis drug development pipeline. Chem. Biol. Drug Des. 86 , 951–960 (2015).
Chao, M. C. & Rubin, E. J. Letting sleeping dos lie: does dormancy play a role in tuberculosis? Annu. Rev. Microbiol. 64 , 293–311 (2010).
Colangeli, R. et al. Bacterial factors that predict relapse after tuberculosis therapy. N. Engl. J. Med. 379 , 823–833 (2018).
Thompson, E. G. et al. Host blood RNA signatures predict the outcome of tuberculosis treatment. Tuberculosis 107 , 48–58 (2017).
Heyckendorf, J. et al. Prediction of anti-tuberculosis treatment duration based on a 22-gene transcriptomic model. Eur. Respir. J. 58 , 2003492 (2021).
Zong, K., Luo, C., Zhou, H., Jiang, Y. & Li, S. Xpert MTB/RIF assay for the diagnosis of rifampicin resistance in different regions: a meta-analysis. BMC Microbiol. 19 , 177 (2019).
Nathavitharana, R. R. et al. Accuracy of line probe assays for the diagnosis of pulmonary and multidrug-resistant tuberculosis: a systematic review and meta-analysis. Eur. Respir. J. 49 , 1601075 (2017).
World Health Organization. Catalogue of mutations in Mycobacterium tuberculosis complex and their association with drug resistance (WHO, 2021).
Gabbassov, E., Moreno-Molina, M., Comas, I., Libbrecht, M. & Chindelevitch, L. SplitStrains, a tool to identify and separate mixed Mycobacterium tuberculosis infections from WGS data. Microb. Genom. 7 , 000607 (2021).
CAS PubMed Central Google Scholar
Cohen, T. et al. Mixed-strain Mycobacterium tuberculosis infections and the implications for tuberculosis treatment and control. Clin. Microbiol. Rev. 25 , 708–719 (2012).
Band, V. I. & Weiss, D. S. Heteroresistance: a cause of unexplained antibiotic treatment failure? PLoS Pathog. 15 , e1007726 (2019).
Ernst, J. D. Mechanisms of M. tuberculosis immune evasion as challenges to TB vaccine design. Cell Host Microbe 24 , 34–42 (2018).
Chai, Q., Wang, L., Liu, C. H. & Ge, B. New insights into the evasion of host innate immunity by Mycobacterium tuberculosis . Cell Mol. Immunol. 17 , 901–913 (2020).
Prideaux, B. et al. The association between sterilizing activity and drug distribution into tuberculosis lesions. Nat. Med. 21 , 1223–1227 (2015).
Sarathy, J. P. & Dartois, V. Caseum: a niche for Mycobacterium tuberculosis drug-tolerant persisters. Clin. Microbiol. Rev. 33 , e00159-19 (2020).
Mashabela, G. T., de Wet, T. J. & Warner, D. F. Mycobacterium tuberculosis metabolism. Microbiol. Spectr. https://doi.org/10.1128/microbiolspec.GPP3-0067-2019 (2019).
Hawn, T. R., Shah, J. A. & Kalman, D. New tricks for old dogs: countering antibiotic resistance in tuberculosis with host-directed therapeutics. Immunol. Rev. 264 , 344–362 (2015).
Lai, R. P. et al. Transcriptomic characterization of tuberculous sputum reveals a host Warburg effect and microbial cholesterol catabolism. mBio 12 , e0176621 (2021).
Larkins-Ford, J. et al. Systematic measurement of combination-drug landscapes to predict in vivo treatment outcomes for tuberculosis. Cell Syst. 12 , 1046–1063.e7 (2021).
Bosch, B. et al. Genome-wide gene expression tuning reveals diverse vulnerabilities of M. tuberculosis . Cell 184 , 4579–4592.e24 (2021).
Rock, J. M. et al. Programmable transcriptional repression in mycobacteria using an orthogonal CRISPR interference platform. Nat. Microbiol. 2 , 16274 (2017).
Cokol, M., Kuru, N., Bicak, E., Larkins-Ford, J. & Aldridge, B. B. Efficient measurement and factorization of high-order drug interactions in Mycobacterium tuberculosis . Sci. Adv. 3 , e1701881 (2017).
Ma, S. et al. Transcriptomic signatures predict regulators of drug synergy and clinical regimen efficacy against tuberculosis. mBio 10 , e02627-19 (2019).
Clemens, D. L. et al. Artificial intelligence enabled parabolic response surface platform identifies ultra-rapid near-universal TB drug treatment regimens comprising approved drugs. PLoS ONE 14 , e0215607 (2019).
Lee, B. Y. et al. Ultra-rapid near universal TB drug regimen identified via parabolic response surface platform cures mice of both conventional and high susceptibility. PLoS ONE 13 , e0207469 (2018).
Band, V. I. et al. Antibiotic combinations that exploit heteroresistance to multiple drugs effectively control infection. Nat. Microbiol. 4 , 1627–1635 (2019).
Peterson, E. J. R., Ma, S., Sherman, D. R. & Baliga, N. S. Network analysis identifies Rv0324 and Rv0880 as regulators of bedaquiline tolerance in Mycobacterium tuberculosis . Nat. Microbiol. 1 , 16078 (2016).
Walter, N. D. et al. Mycobacterium tuberculosis precursor rRNA as a measure of treatment-shortening activity of drugs and regimens. Nat. Commun. 12 , 2899 (2021).
Gideon, H. P. et al. Multimodal profiling of lung granulomas reveals cellular correlates of tuberculosis control. bioRxiv https://doi.org/10.1101/2020.10.24.352492 (2021). Preprint at.
Dinkele, R. et al. Capture and visualization of live Mycobacterium tuberculosis bacilli from tuberculosis patient bioaerosols. PLoS Pathog. 17 , e1009262 (2021).
Berry, M. P. et al. An interferon-inducible neutrophil-driven blood transcriptional signature in human tuberculosis. Nature 466 , 973–977 (2010).
Wells, G. et al. μCT analysis of the human tuberculous lung reveals remarkable heterogeneity in 3D granuloma morphology. Am. J. Respir. Crit. Care Med. 204 , 583–595 (2021).
Chen, R. Y. et al. Radiological and functional evidence of the bronchial spread of tuberculosis: an observational analysis. Lancet Microbe 2 , e518–e526 (2021).
Dartois, V. & Dick, T. A ginger root or plum model for the TB “granuloma”? Am. J. Respir. Crit. Care Med. 204 , 505–507 (2021).
Hunter, R. L. The pathogenesis of tuberculosis — the Koch phenomenon reinstated. Pathogens 9 , 813 (2020).
Libardo, J., Boshoff, H. I. & Barry, C. E. 3rd The present state of the tuberculosis drug development pipeline. Curr. Opin. Pharmacol. 42 , 81–94 (2018).
Article PubMed Central Google Scholar
Bartelink, I. H. et al. New paradigm for translational modeling to predict long-term tuberculosis treatment response. Clin. Transl. Sci. 10 , 366–379 (2017).
Pienaar, E. et al. A computational tool integrating host immunity with antibiotic dynamics to study tuberculosis treatment. J. Theor. Biol. 367 , 166–179 (2015).
Ernest, J. et al. Development of new tuberculosis drugs: translation to regimen composition for drug-sensitive and multidrug-resistant tuberculosis. Annu. Rev. Pharmacol. Toxicol. 61 , 495–516 (2020).
Mudde, S. E. et al. Predictive modeling to study the treatment-shortening potential of novel tuberculosis drug regimens, towards bundling of preclinical data. J. Infect. Dis. https://doi.org/10.1093/infdis/jiab101 (2021).
Dooley, K. E., Hanna, D., Mave, V., Eisenach, K. & Savic, R. M. Advancing the development of new tuberculosis treatment regimens: the essential role of translational and clinical pharmacology and microbiology. PLoS Med. 16 , e1002842 (2019).
Lenaerts, A., Barry, C. E. 3rd & Dartois, V. Heterogeneity in tuberculosis pathology, microenvironments and therapeutic responses. Immunol. Rev. 264 , 288–307 (2015).
Nuermberger, E. L. Preclinical efficacy testing of new drug candidates. Microbiol. Spectr. https://doi.org/10.1128/microbiolspec.TBTB2-0034-2017 (2017).
Via, L. E. et al. A sterilizing tuberculosis treatment regimen is associated with faster clearance of bacteria in cavitary lesions in marmosets. Antimicrob. Agents Chemother. 59 , 4181–4189 (2015).
White, A. G. et al. Analysis of 18FDG PET/CT imaging as a tool for studying Mycobacterium tuberculosis infection and treatment in non-human primates. J. Vis. Exp. 127 , 56375 (2017).
Google Scholar
Xie, Y. L. et al. Fourteen-day PET/CT imaging to monitor drug combination activity in treated individuals with tuberculosis. Sci. Transl Med. 13 , eabd7618 (2021).
Lienhardt, C. et al. Advances in clinical trial design: weaving tomorrow’s TB treatments. PLoS Med. 17 , e1003059 (2020).
Davies, G., Boeree, M., Hermann, D. & Hoelscher, M. Accelerating the transition of new tuberculosis drug combinations from phase II to phase III trials: new technologies and innovative designs. PLoS Med. 16 , e1002851 (2019).
Strydom, N. et al. Tuberculosis drugs’ distribution and emergence of resistance in patient’s lung lesions: a mechanistic model and tool for regimen and dose optimization. PLoS Med. 16 , e1002773 (2019).
Lewis, K. & Shan, Y. Why tolerance invites resistance. Science 355 , 796 (2017).
Schrader, S. M., Vaubourgeix, J. & Nathan, C. Biology of antimicrobial resistance and approaches to combat it. Sci. Transl Med. 12 , eaaz6992 (2020).
Hicks, N. D. et al. Clinically prevalent mutations in Mycobacterium tuberculosis alter propionate metabolism and mediate multidrug tolerance. Nat. Microbiol. 3 , 1032–1042 (2018).
Zhu, J. H. et al. Rifampicin can induce antibiotic tolerance in mycobacteria via paradoxical changes in rpoB transcription. Nat. Commun. 9 , 4218 (2018).
Bellerose, M. M. et al. Common variants in the glycerol kinase gene reduce tuberculosis drug efficacy. mBio 10 , e00663-19 (2019).
Safi, H. et al. Phase variation in Mycobacterium tuberculosis glpK produces transiently heritable drug tolerance. Proc. Natl Acad. Sci. USA 116 , 19665–19674 (2019).
Lee, J. J. et al. Transient drug-tolerance and permanent drug-resistance rely on the trehalose-catalytic shift in Mycobacterium tuberculosis . Nat. Commun. 10 , 2928 (2019).
Lim, J. et al. Phosphoenolpyruvate depletion mediates both growth arrest and drug tolerance of Mycobacterium tuberculosis in hypoxia. Proc. Natl Acad. Sci. USA 118 , e2105800118 (2021).
Baek, S. H., Li, A. H. & Sassetti, C. M. Metabolic regulation of mycobacterial growth and antibiotic sensitivity. PLoS Biol. 9 , e1001065 (2011).
Martini, M. C. et al. Loss of RNase J leads to multi-drug tolerance and accumulation of highly structured mRNA fragments in Mycobacterium tuberculosis . Preprint at bioRxiv https://doi.org/10.1101/2022.02.13.480260 (2022).
Zheng, H. et al. Inhibitors of Mycobacterium tuberculosis DosRST signaling and persistence. Nat. Chem. Biol. 13 , 218–225 (2017).
Chaudhuri, S. et al. Kasugamycin potentiates rifampicin and limits emergence of resistance in Mycobacterium tuberculosis by specifically decreasing mycobacterial mistranslation. eLife 7 , e36782 (2018).
Liu, Y. et al. Immune activation of the host cell induces drug tolerance in Mycobacterium tuberculosis both in vitro and in vivo. J. Exp. Med. 213 , 809–825 (2016).
Prosser, G. et al. The bacillary and macrophage response to hypoxia in tuberculosis and the consequences for T cell antigen recognition. Microbes Infect. 19 , 177–192 (2017).
Tan, S., Sukumar, N., Abramovitch, R. B., Parish, T. & Russell, D. G. Mycobacterium tuberculosis responds to chloride and pH as synergistic cues to the immune status of its host cell. PLoS Pathog. 9 , e1003282 (2013).
Datta, M. et al. Anti-vascular endothelial growth factor treatment normalizes tuberculosis granuloma vasculature and improves small molecule delivery. Proc. Natl Acad. Sci. USA 112 , 1827–1832 (2015).
Boldrin, F., Provvedi, R., Cioetto Mazzabo, L., Segafreddo, G. & Manganelli, R. Tolerance and persistence to drugs: a main challenge in the fight against Mycobacterium tuberculosis . Front. Microbiol. 11 , 1924 (2020).
Gessner, S. et al. The mycobacterial mutasome: composition and recruitment in live cells. bioRxiv https://doi.org/10.1101/2021.11.16.468908 (2021). Preprint at.
Ragheb, M. N. et al. Inhibiting the evolution of antibiotic resistance. Mol. Cell 73 , 157–165.e5 (2019).
Awasthi, D. & Freundlich, J. S. Antimycobacterial metabolism: illuminating Mycobacterium tuberculosis biology and drug discovery. Trends Microbiol. 25 , 756–767 (2017).
Varaksa, T. et al. Metabolic fate of human immunoactive sterols in Mycobacterium tuberculosis . J. Mol. Biol. 433 , 166763 (2021).
Remm, S., Earp, J. C., Dick, T., Dartois, V. & Seeger, M. A. Critical discussion on drug efflux in Mycobacterium tuberculosis . FEMS Microbiol. Rev. 46 , fuab050 (2022).
Lee, R. E. et al. Spectinamides: a new class of semisynthetic antituberculosis agents that overcome native drug efflux. Nat. Med. 20 , 152–158 (2014).
Laws, M., Jin, P. & Rahman, K. M. Efflux pumps in Mycobacterium tuberculosis and their inhibition to tackle antimicrobial resistance. Trends Microbiol. 30 , 57–68 (2022).
World Health Organization. The end TB strategy (WHO, 2015).
Franzblau, S. G. et al. Comprehensive analysis of methods used for the evaluation of compounds against Mycobacterium tuberculosis . Tuberculosis 92 , 453–488 (2012).
Zhang, M. et al. Streptomycin-starved Mycobacterium tuberculosis 18b, a drug discovery tool for latent tuberculosis. Antimicrob. Agents Chemother. 56 , 5782–5789 (2012).
Mitchison, D. A. & Coates, A. R. Predictive in vitro models of the sterilizing activity of anti-tuberculosis drugs. Curr. Pharm. Des. 10 , 3285–3295 (2004).
Bassett, I. M. et al. Detection of inhibitors of phenotypically drug-tolerant Mycobacterium tuberculosis using an in vitro bactericidal screen. J. Microbiol. 51 , 651–658 (2013).
Darby, C. M. et al. Whole cell screen for inhibitors of pH homeostasis in Mycobacterium tuberculosis . PLoS ONE 8 , e68942 (2013).
Early, J. et al. Identification of compounds with pH-dependent bactericidal activity against Mycobacterium tuberculosis . ACS Infect. Dis. 5 , 272–280 (2019).
Gouzy, A., Healy, C., Black, K. A., Rhee, K. Y. & Ehrt, S. Growth of Mycobacterium tuberculosis at acidic pH depends on lipid assimilation and is accompanied by reduced GAPDH activity. Proc. Natl Acad. Sci. USA 118 , e2024571118 (2021).
Grant, S. S. et al. Identification of novel inhibitors of nonreplicating Mycobacterium tuberculosis using a carbon starvation model. ACS Chem. Biol. 8 , 2224–2234 (2013).
Dahl, J. L. et al. The role of RelMtb-mediated adaptation to stationary phase in long-term persistence of Mycobacterium tuberculosis in mice. Proc. Natl Acad. Sci. USA 100 , 10026–10031 (2003).
Betts, J. C., Lukey, P. T., Robb, L. C., McAdam, R. A. & Duncan, K. Evaluation of a nutrient starvation model of Mycobacterium tuberculosis persistence by gene and protein expression profiling. Mol. Microbiol. 43 , 717–731 (2002).
Loebel, R. O., Shorr, E. & Richardson, H. B. The influence of foodstuffs upon the respiratory metabolism and growth of human tubercle bacilli. J. Bacteriol. 26 , 139–166 (1933).
Cho, S. H. et al. Low-oxygen-recovery assay for high-throughput screening of compounds against nonreplicating Mycobacterium tuberculosis . Antimicrob. Agents Chemother. 51 , 1380–1385 (2007).
Wayne, L. G. & Hayes, L. G. An in vitro model for sequential study of shiftdown of Mycobacterium tuberculosis through two stages of nonreplicating persistence. Infect. Immun. 64 , 2062–2069 (1996).
Boon, C. & Dick, T. Mycobacterium bovis BCG response regulator essential for hypoxic dormancy. J. Bacteriol. 184 , 6760–6767 (2002).
Via, L. E. et al. Tuberculous granulomas are hypoxic in guinea pigs, rabbits, and non-human primates. Infect. Immun. 76 , 2333–2340 (2008).
Lovewell, R. R., Sassetti, C. M. & VanderVen, B. C. Chewing the fat: lipid metabolism and homeostasis during M. tuberculosis infection. Curr. Opin. Microbiol. 29 , 30–36 (2016).
Pandey, A. K. & Sassetti, C. M. Mycobacterial persistence requires the utilization of host cholesterol. Proc. Natl Acad. Sci. USA 105 , 4376–4380 (2008).
Garton, N. J. et al. Cytological and transcript analyses reveal fat and lazy persister-like bacilli in tuberculous sputum. PLoS Med. 5 , e75 (2008).
Kim, M. J. et al. Caseation of human tuberculosis granulomas correlates with elevated host lipid metabolism. EMBO Mol. Med. 2 , 258–274 (2010).
Gold, B., Warrier, T. & Nathan, C. A multistress model for high throughput screening against nonreplicating Mycobacterium tuberculosis . Methods Mol. Biol. 2314 , 611–635 (2021).
Christophe, T., Ewann, F., Jeon, H. K., Cechetto, J. & Brodin, P. High-content imaging of Mycobacterium tuberculosis -infected macrophages: an in vitro model for tuberculosis drug discovery. Future Med. Chem. 2 , 1283–1293 (2010).
Song, O. R. et al. Phenotypic assays for Mycobacterium tuberculosis infection. Cytometry A 91 , 983–994 (2017).
Chakraborty, P., Bajeli, S., Kaushal, D., Radotra, B. D. & Kumar, A. Biofilm formation in the lung contributes to virulence and drug tolerance of Mycobacterium tuberculosis . Nat. Commun. 12 , 1606 (2021).
Ackart, D. F. et al. Expression of antimicrobial drug tolerance by attached communities of Mycobacterium tuberculosis . Pathog. Dis. 70 , 359–369 (2014).
Ojha, A. K. et al. Growth of Mycobacterium tuberculosis biofilms containing free mycolic acids and harbouring drug-tolerant bacteria. Mol. Microbiol. 69 , 164–174 (2008).
Mukamolova, G. V., Turapov, O., Malkin, J., Woltmann, G. & Barer, M. R. Resuscitation-promoting factors reveal an occult population of tubercle bacilli in sputum. Am. J. Respir. Crit. Care Med. 181 , 174–180 (2010).
Lipworth, S. et al. Defining dormancy in mycobacterial disease. Tuberculosis 99 , 131–142 (2016).
Shetye, G. S., Franzblau, S. G. & Cho, S. New tuberculosis drug targets, their inhibitors, and potential therapeutic impact. Transl. Res. 220 , 68–97 (2020).
Singh, R. et al. PA-824 kills nonreplicating Mycobacterium tuberculosis by intracellular NO release. Science 322 , 1392–1395 (2008).
Robertson, G. T. et al. Comparative analysis of pharmacodynamics in the C3HeB/FeJ mouse tuberculosis model for DprE1 inhibitors TBA-7371, PBTZ169, and OPC-167832. Antimicrob. Agents Chemother. 65 , e0058321 (2021).
Tenero, D. et al. First-time-in-human study and prediction of early bactericidal activity for GSK3036656, a potent leucyl-tRNA synthetase inhibitor for tuberculosis treatment. Antimicrob. Agents Chemother. 63 , e00240-19 (2019).
Zumla, A., Nahid, P. & Cole, S. T. Advances in the development of new tuberculosis drugs and treatment regimens. Nat. Rev. Drug Discov. 12 , 388–404 (2013).
Rawson, T. M. et al. Optimizing antimicrobial use: challenges, advances and opportunities. Nat. Rev. Microbiol. 19 , 747–758 (2021).
Roemhild, R., Bollenbach, T. & Andersson, D. I. The physiology and genetics of bacterial responses to antibiotic combinations. Nat. Rev. Microbiol. https://doi.org/10.1038/s41579-022-00700-5 (2022).
Tyers, M. & Wright, G. D. Drug combinations: a strategy to extend the life of antibiotics in the 21st century. Nat. Rev. Microbiol. 17 , 141–155 (2019).
Robertson, B. D. et al. Detection and treatment of subclinical tuberculosis. Tuberculosis 92 , 447–452 (2012).
Vernon, A. & Bishai, W. Modeling treatment of latent tuberculosis: shortening the leap of faith? Am. J. Respir. Crit. Care Med. 201 , 405–406 (2020).
Bucsan, A. N., Mehra, S., Khader, S. A. & Kaushal, D. The current state of animal models and genomic approaches towards identifying and validating molecular determinants of Mycobacterium tuberculosis infection and tuberculosis disease. Pathog. Dis. 77 , ftz037 (2019).
Oh, C. E. & Menzies, D. Four months of rifampicin monotherapy for latent tuberculosis infection in children. Clin. Exp. Pediatr. https://doi.org/10.3345/cep.2021.01186 (2021).
Fox, G. J. et al. Preventing the spread of multidrug-resistant tuberculosis and protecting contacts of infectious cases. Clin. Microbiol. Infect. 23 , 147–153 (2017).
Download references
Acknowledgements
The authors thank B. B. Aldridge and T. Dick for stimulating discussions. Support for work in the authors’ laboratories was provided by grants INV-009173 and INV-004704 from the Bill and Melinda Gates Foundation and grant U19AI142735 from the National Institutes of Health.
Author information
Authors and affiliations.
Center for Discovery and Innovation, and Hackensack Meridian School of Medicine, Department of Medical Sciences, Hackensack Meridian Health, Nutley, NJ, USA
Véronique A. Dartois
Harvard T.H. Chan School of Public Health, Department of Immunology and Infectious Diseases, Boston, MA, USA
Eric J. Rubin
You can also search for this author in PubMed Google Scholar
Contributions
V. A. D. researched data for the article. Both authors contributed substantially to discussion of the content, wrote the article, and edited and reviewed the manuscript before submission.
Corresponding author
Correspondence to Véronique A. Dartois .
Ethics declarations
Competing interests.
The authors declare no competing interests.
Peer review
Peer review information.
Nature Reviews Microbiology thanks David Sherman, Mel Spigelman and the other, anonymous, reviewer(s) for their contribution to the peer review of this work.
Additional information
Publisher’s note.
Springer Nature remains neutral with regard to jurisdictional claims in published maps and institutional affiliations.
Related links
ClinicalTrials.gov: https://clinicaltrials.gov
The World Bank database: https://data.worldbank.org/indicator/SH.TBS.INCD?end=2020&locations=ZA-RU-CN&start=2000&view=chart
WHO tuberculosis: https://www.who.int/news-room/fact-sheets/detail/tuberculosis
Working Group on New TB Drugs: https://www.newtbdrugs.org/pipeline/clinical
Supplementary information
Supplementary information.
Disease that occurs in individuals infected with Mycobacterium tuberculosis and present symptoms that include cough, weight loss, night sweats and fever.
Active disease characterized by the presence of open pulmonary cavities in the lung parenchyma, composed of a wall filled with necrotic debris and high bacterial burden, leading to poor treatment outcome and increased person-to-person transmission.
Increased survival of bacterial cells in the presence of drug, generally not driven by genetic mutations but due to physiological or metabolic adaptations that lead to decreased growth rate upon encountering specific environmental conditions.
The subpopulation of bacteria that survives the bactericidal action of antibiotics but is genetically identical to susceptible bacteria and appears to be non-replicating or slowly growing in response to various stresses.
(MIC). The lowest drug concentration that inhibits growth.
The minimum inhibitory concentration (MIC) above which an antimicrobial agent is considered to have a low probability of treatment success in the clinic.
Interactions that occur when the combined effect of two drugs is greater than the sum of each drug’s individual activity.
Mycobacterium tuberculosis infection presenting with mild or no symptoms, where low bacterial burden persists in a quiescent state, can be detected by conventional immune-based assays and can be intermittently culture-positive but sputum smear-negative due to the low bacillary load.
The protein components that assemble at a DNA lesion in the presence of a stalled replication complex to mediate translesion DNA synthesis in a mutagenic manner.
Rights and permissions
Reprints and permissions
About this article
Cite this article.
Dartois, V.A., Rubin, E.J. Anti-tuberculosis treatment strategies and drug development: challenges and priorities. Nat Rev Microbiol 20 , 685–701 (2022). https://doi.org/10.1038/s41579-022-00731-y
Download citation
Accepted : 23 March 2022
Published : 27 April 2022
Issue Date : November 2022
DOI : https://doi.org/10.1038/s41579-022-00731-y
Share this article
Anyone you share the following link with will be able to read this content:
Sorry, a shareable link is not currently available for this article.
Provided by the Springer Nature SharedIt content-sharing initiative
This article is cited by
Trends in the availability and prices of quality-assured tuberculosis drugs: a systematic analysis of global drug facility product catalogs from 2001 to 2024.
- Stefan Kohler
- Nicolas Paul
Globalization and Health (2024)
- Véronique Dartois
- Thomas Dick
Nature Reviews Drug Discovery (2024)
Discovery of potent antimycobacterial agents targeting lumazine synthase (RibH) of Mycobacterium tuberculosis
- Monica Singh
- Anannya Dhanwal
- Ruchi Jain Dey
Scientific Reports (2024)
TcrXY is an acid-sensing two-component transcriptional regulator of Mycobacterium tuberculosis required for persistent infection
- Miljan Stupar
- Nicholas P. West
Nature Communications (2024)
A spotlight on the tuberculosis epidemic in South Africa
Quick links.
- Explore articles by subject
- Guide to authors
- Editorial policies
Sign up for the Nature Briefing: Translational Research newsletter — top stories in biotechnology, drug discovery and pharma.


COMMENTS
Introduction. Tuberculosis (TB) represents a major global health threat that, despite being preventable and treatable, is the 13th leading cause of death worldwide and the second leading infectious killer after coronavirus disease 2019 (COVID-19) [1, 2].In the past decades, the TB burden has been slowly decreasing; however, with the emergence of COVID-19 and the current political conflicts ...
Tuberculosis (TB), a communicable disease mainly due to infection with Mycobacterium tuberculosis (MTB), remains a major public health problem afflicts approximately 10 million people worldwide ...
Tuberculosis (TB) is a major cause of morbidity and mortality worldwide. It is estimated that 25% of the world's population are infected with Mycobacterium tuberculosis, with a 5-10% lifetime risk of progression into TB disease. Early recognition of TB disease and prompt detection of drug resistance are essential to halting its global burden.
Tuberculosis (TB) encompasses a vast amount of information about a common disease that is challenging to diagnose, treat, and prevent. The following quote by S.T. Cole provides a perspective on the importance of this disease: "More human lives have been lost to tuberculosis than to any other disease."[1] Before the SARS-CoV-2 pandemic, Mycobacterium tuberculosis (Mtb) was the most ...
Patients with highly drug-resistant forms of tuberculosis have limited treatment options and historically have had poor outcomes. In an open-label, single-group study in which follow-up is ongoing ...
Tuberculosis remains the leading cause of death from an infectious disease among adults worldwide, with more than 10 million people becoming newly sick from tuberculosis each year. Advances in diagnosis, including the use of rapid molecular testing and whole-genome sequencing in both sputum and non-sputum samples, could change this situation. Although little has changed in the treatment of ...
Tuberculosis (TB) is an airborne infectious disease caused by organisms of the Mycobacterium tuberculosis complex. Although primarily a pulmonary pathogen, M. tuberculosis can cause disease in ...
In 2010, 8.8 million people developed tuberculosis—a contagious bacterial infection—and 1.4 million people died from the disease. Mycobacterium tuberculosis, the bacterium that causes tuberculosis, is spread in airborne droplets when people with the disease cough or sneeze and usually infects the lungs (pulmonary tuberculosis). The ...
Tuberculosis Research and Treatment publishes original research articles and review articles related to all aspects of tuberculosis, ... Assessment of Smear‐Positive Pulmonary Tuberculosis and Associated Factors among Patients Visiting Health Facilities of Gedeo Zone, Southern Ethiopia: A Cross‐Sectional Study.
The WHO reported an alarming 10.6 million cases of tuberculosis in 2021 [1].Indirect evidence suggests that one fourth of the world's population is estimated to be infected with Mycobacterium tuberculosis [2], and 5-10% of those infected develop tuberculosis in their lifetime [3].There is a paradigm shift in our understanding of tuberculosis natural history—instead of perpetuating a binary ...
Tuberculosis is highly prevalent among the low socioeconomic section of the population and marginalized sections of the community. In India, National strategic plan (2017-2025) has a national goal of elimination of tuberculosis by 2025. It requires increased awareness and understanding of Tuberculosis. In this review article history, taxonomy ...
Tuberculosis (TB) is a major cause of morbidity and mortality worldwide. It is estimated that 25% of the world's population are infected with Mycobacterium tuberculosis , with a 5-10% lifetime risk of progression into TB disease. Early recognition of TB disease and prompt detection of drug resistance are essential to halting its global burden. Culture, direct microscopy, biomolecular tests ...
Box 1. Pulmonary tuberculosis (TB) remains a major cause of morbidity and mortality worldwide, with about one-third of the world's population infected. 1 Between 10% and 20% of those infected will progress to active TB, posing a serious health threat. The remaining patients will have latent TB, which can advance to active infection in times of ...
Laneke Luies completed her Ph.D., entitled "Characterising Tuberculosis and Treatment Failure Thereof Using Metabolomics," in 2017 at the North-West University (NWU) in Potchefstroom, South Africa. Thereafter, Dr. Luies started two postdoctoral research fellowships at the University of Cape Town (2017) and the NWU (2018) and is currently employed at the Focus Area: Human Metabolomics (at ...
RSS Feed. Tuberculosis (TB) is an infectious disease caused by strains of bacteria known as mycobacteria. The disease most commonly affects the lungs and can be fatal if not treated. However, most ...
Guidelines on the treatment of tuberculosis (TB) have essentially remained the same for the past 35 years, but are now starting to change. Ongoing clinical trials will hopefully transform the landscape for treatment of drug sensitive TB, drug resistant TB, and latent TB infection. Multiple trials are evaluating novel agents, repurposed agents, adjunctive host directed therapies, and novel ...
However, this regimen should only be administered under operational research conditions and when BDQ and linezolid have not been previously used . PMD, ... The Echo of Pulmonary Tuberculosis: Mechanisms of Clinical Symptoms and Other Disease-Induced Systemic Complications. Clin. Microbiol. Rev. 2020; 33:e00036-20. doi: 10.1128/CMR.00036-20.
Objective The proportion of COVID-19 patients having active pulmonary tuberculosis, and its impact on COVID-19 related patient outcomes, is not clear. We conducted this systematic review to evaluate the proportion of patients with active pulmonary tuberculosis among COVID-19 patients, and to assess if comorbid pulmonary tuberculosis worsens clinical outcomes in these patients. Methods We ...
Abstract and Figures. Tuberculosis Is a major bluster to humanity resist to progress in health-care systems and the widespread weapon of TB control programs. The World Health Organization (WHO ...
Abstract. Tuberculosis (TB) remains one of the deadliest infectious diseases responsible for millions of deaths annually across the world. In this paper we present a general overview of TB ...
A retrospective study of sputum conversion in sputum positive 1782 pulmonary tuberculosis (PTB) was conducted from 2021 to 2022 in Beijing, China. ... Research articles News & Comment Collections ...
Tuberculosis (TB) remains a significant global health concern, necessitating accurate and timely diagnostic methods. This study aimed to conduct a systematic review and meta-analysis to assess the diagnostic accuracy of Truenat assays for both pulmonary TB (PTB) and extrapulmonary TB (EPTB).
Vyawahare C, Mukhida S, Khan S, et al. Assessment of risk factors associated with drug-resistant tuberculosis in pulmonary tuberculosis patients. Indian J TB. 2023;71:S44-S51. ... Sage Research Methods Supercharging research opens in new tab; Sage Video Streaming knowledge opens in new tab;
Epidemiological clues to diagnosis. Among immigrants to the West from the Indian subcontinent, sub-Saharan Africa, South East Asia, the Baltic states and Russia (especially if they were previously imprisoned 4), the prevalence of tuberculosis is much higher than among the native white population. 2,5 In the native population, tuberculosis is most commonly found among people living in poor ...
1.Introduction. Tuberculosis caused by Mycobacterium tuberculosis complex (MTBC) remains a global health emergency and it is the second deadliest infectious disease caused by a single infectious agent after COVID-19. Globally, it is estimated that over 10 million new cases of TB occurred in 2022 and a quarter of these were from Africa [1].Moreover, a worldwide estimate indicates that, 1.3 ...
This article is part of the Research Topic Innovative Tuberculosis Case Finding Interventions: Lessons From the Field View all 9 articles. Spatiotemporal analysis of tuberculosis in the Hunan Province, China, 2014-2022. ... Background: Pulmonary tuberculosis (PTB) is a major infectious disease that threatens human health. China is a high ...
A CMV-vectored vaccine (RhCMV/TB) led to a 68% reduction in M. tuberculosis infection and disease compared to unvaccinated controls in rhesus macaques 20. Notably, 14 out of 34 vaccinated animals ...
Rasmussen aneurysm, a rare complication of cavitatory tuberculosis (TB), manifests as an aneurysmal dilatation of the pulmonary arterial branch, occurring in approximately one out of 14,000 patients (0.007%) [].The associated hemoptysis can pose significant risks to life and is predominantly managed through embolization techniques, given the complexities associated with surgical approaches [].
Primary pulmonary TB should be distinguished from postprimary pulmonary TB, which is the most frequent TB manifestation in adults. The classic clinical features of pulmonary TB include chronic cough, sputum production, appetite loss, weight loss, fever, night sweats, and hemoptysis ( Lawn and Zumla 2011 ). Someone presenting with any of these ...
Despite two decades of intensified research to understand and cure tuberculosis disease, biological uncertainties remain and hamper progress. However, owing to collaborative initiatives including ...